Key Factors Influencing Heat Transfer and Combustion Efficiency in Industrial Coal-Fired Boilers
Industrial coal-fired boilers remain vital for heavy-duty heat and steam applications, but their efficiency is highly dependent on combustion control and heat transfer performance. If either is suboptimal, it leads to incomplete combustion, excess fuel use, high emissions, and reduced steam output. For plant operators, understanding the core factors that influence these efficiencies is key to maximizing output, lowering fuel costs, and ensuring long-term operational stability.
The heat transfer and combustion efficiency in industrial coal-fired boilers are influenced by factors such as fuel type and quality, air-fuel ratio, combustion temperature, boiler design, ash deposition, slagging behavior, and cleanliness of heat exchange surfaces. Efficient combustion ensures full fuel burn with minimal unburned carbon, while effective heat transfer allows maximum energy absorption from flue gases into steam. Neglecting either aspect results in energy losses, fouling, and increased emissions.
Let’s examine the critical technical variables that affect the overall efficiency of coal-fired boiler systems.
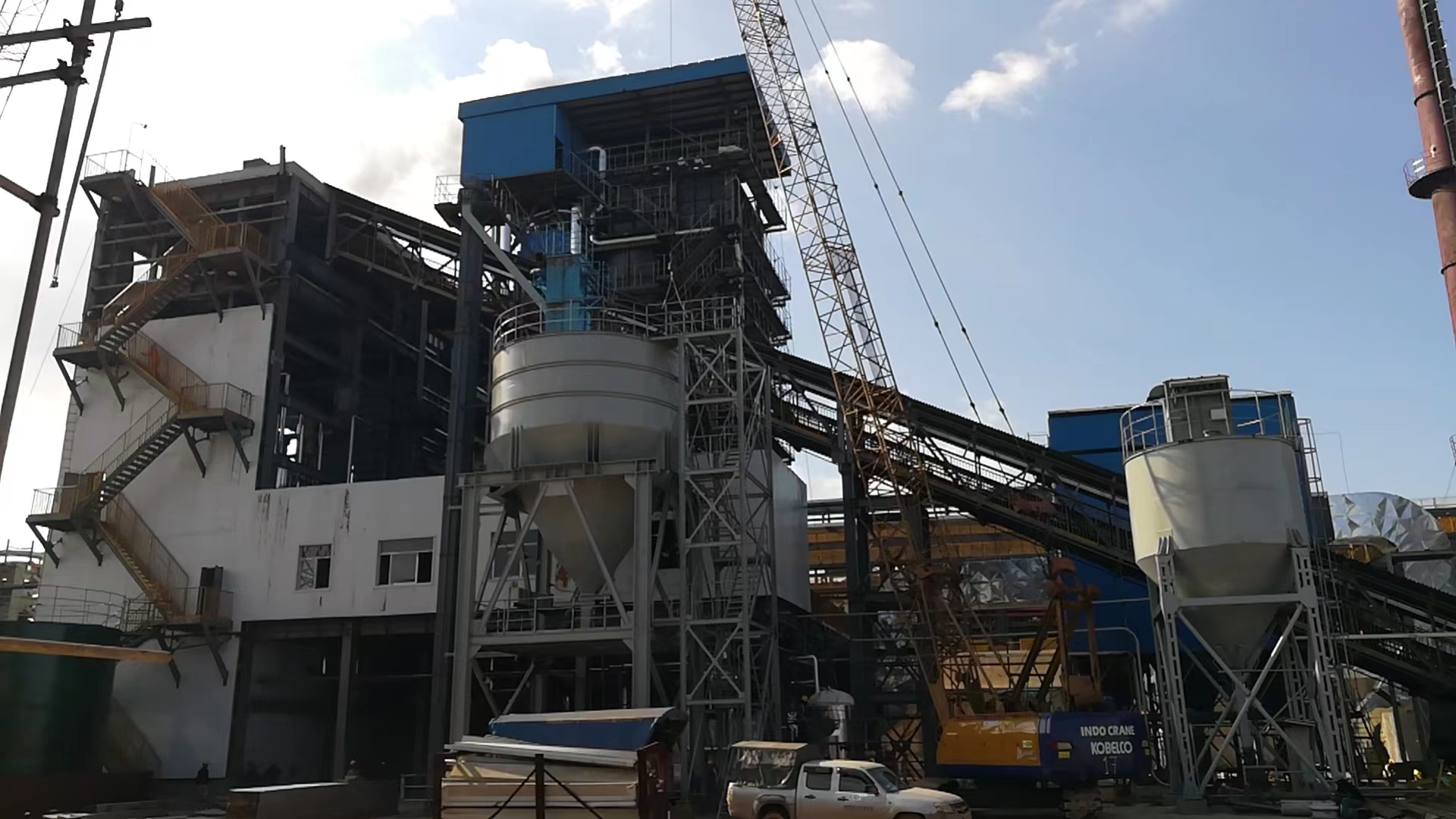
How Does Coal Type (Calorific Value, Ash, Moisture) Impact Combustion Performance?
Coal is still a widely used fuel in industrial steam boiler systems, especially in power generation, cement, and metallurgy sectors. However, not all coals are created equal. Variations in calorific value, ash content, and moisture levels dramatically influence combustion performance, heat transfer efficiency, emissions, and operational stability. If coal is poorly matched to boiler design—or if its properties vary without adjustment—combustion becomes unstable, heat output declines, slagging increases, and emissions exceed limits. Understanding coal characteristics is therefore crucial for optimizing boiler performance and regulatory compliance.
Coal type—defined by calorific value, ash content, and moisture—has a significant impact on combustion performance in steam boilers. Low-calorific coals require more fuel per unit energy, increasing flue gas volume and reducing efficiency. High ash content lowers heat transfer, causes slagging and erosion, and increases particulate emissions. Moisture-rich coal absorbs heat for evaporation, reducing flame temperature and combustion stability. Matching coal properties to boiler design and adjusting combustion parameters accordingly are essential to maintain efficient, clean, and stable operation.
Each property influences not just combustion—but the entire thermal and emissions balance of the boiler system.
Coal type, including calorific value, ash, and moisture, significantly impacts boiler combustion performance.True
These properties affect energy release, flame stability, ash fouling, emissions, and the efficiency of heat transfer and combustion control.
🔍 Key Combustion-Influencing Coal Properties
Property | Definition | Effect on Combustion Performance |
---|---|---|
Calorific Value (CV) | Heat energy per kg of coal (kcal/kg) | Lower CV = more fuel needed, higher stack loss |
Ash Content (%) | Inert mineral matter that doesn’t burn | High ash = more fouling, erosion, lower efficiency |
Moisture (%) | Water in the coal (inherent + surface) | High moisture = flame quenching, higher unburnt fuel |
Volatile Matter (%) | Compounds that vaporize and burn off first | Impacts flame stability and ignition time |
Fixed Carbon (%) | Carbon content available for sustained burn | Controls heat release duration and flame length |
📏 Comparative Properties of Different Coal Types
Coal Type | Calorific Value (kcal/kg) | Ash (%) | Moisture (%) | Volatile Matter (%) |
---|---|---|---|---|
Bituminous | 5,000–6,500 | 10–20 | 4–8 | 20–35 |
Sub-bituminous | 4,000–5,000 | 15–25 | 10–15 | 25–40 |
Lignite | 2,500–3,800 | 20–40 | 20–55 | 35–50 |
Anthracite | 6,500–7,000 | 8–15 | 2–5 | <10 |
→ Lower-grade coals like lignite and high-ash sub-bituminous types require more fuel and more controls.
🔧 Impact of Calorific Value on Boiler Operation
Low CV Coal (e.g., Lignite) | High CV Coal (e.g., Bituminous) |
---|---|
Requires more kg of coal per ton of steam | Less fuel needed → smaller ash and gas volume |
Increases flue gas volume | Less fan power required |
Low flame temperature | Higher combustion stability |
Larger furnace required | More compact design possible |
→ Combustion tuning must adjust air-to-fuel ratio and burner settings to match energy output.
🌫 Effect of Ash Content on Heat Transfer and Fouling
Ash Type | Effect on Boiler |
---|---|
High ash (>25%) | Causes slagging, erosion, PM emission |
Sticky ash (low fusion point) | Forms clinkers on furnace wall |
Fine fly ash | Increases PM load on ESP/baghouse |
Quartz-rich ash | Tube abrasion, high wear rates |
Impact Area | Operational Consequence |
---|---|
Superheater Tubes | Fouling → reduced heat absorption |
Economizer | Plugging → pressure drop rise |
Air Preheater | Ash sticking → heat recovery loss |
ESP/Baghouse | Increased load → frequent cleaning |
→ High ash requires stronger soot blowing and ash handling systems.
💧 Moisture Content and Combustion Efficiency
Moisture (%) | Effect on Combustion |
---|---|
<8% | High flame temp, stable combustion |
10–20% | Flame cooling, reduced burn efficiency |
>30% | Delayed ignition, CO spike, incomplete combustion |
Key Impacts | Efficiency Reduction |
---|---|
Steam loss in stack gas | +4–8% energy loss |
Latent heat used for evaporation | Less heat for steam |
Flame instability | ↑ CO, ↑ unburned carbon |
→ Using pre-drying systems or selecting low-moisture fuel can significantly improve performance.
🧪 Case Study – Sub-Bituminous Coal in a 40 TPH Boiler
Initial Fuel: 5,000 kcal/kg, 28% ash, 12% moisture
Problems:
Stack temp = 285°C
High soot loading
PM emissions > 90 mg/Nm³
Interventions:
Tuning of secondary air
More frequent soot blowing
Added economizer ash cleaning
Results:
Efficiency improved from 75.2% to 81.4%
Stack temp dropped to 220°C
PM reduced to 38 mg/Nm³
📈 Coal Property vs. Boiler Performance Summary Table
Coal Property | Desired Range | Performance Impact |
---|---|---|
Calorific Value | >5,000 kcal/kg | More heat per kg = higher efficiency |
Ash Content | <20% | Less fouling and erosion |
Moisture | <10% | Higher combustion temp, better burnout |
Volatile Matter | 20–35% | Improves ignition, reduces startup time |
Fixed Carbon | 50–70% | Sustains heat release |
→ Fuel testing and characterization must be regular and batch-specific.
🔍 Operational Adjustments Based on Coal Properties
Adjustment Area | Tuning Parameter |
---|---|
Air-to-Fuel Ratio | Reduce excess air for low-moisture fuels |
Burner Configuration | Match flame length to coal burn rate |
Grate Speed (for stokers) | Slow for low CV fuels to allow full burnout |
Soot Blower Schedule | Increase for high ash fuels |
Ash Removal Cycle | Increase hopper discharge for sticky ash |
Smart control systems can dynamically adjust based on coal input variability.
In conclusion, coal type—through its calorific value, ash content, and moisture level—has a profound effect on boiler combustion performance. High-ash, low-CV, and moisture-rich coals require more energy, more controls, and more maintenance. Understanding these characteristics and incorporating them into boiler design and operational tuning is essential for achieving optimal efficiency, reliability, and environmental compliance. Your fuel isn’t just feeding the fire—it’s defining how well your boiler performs.
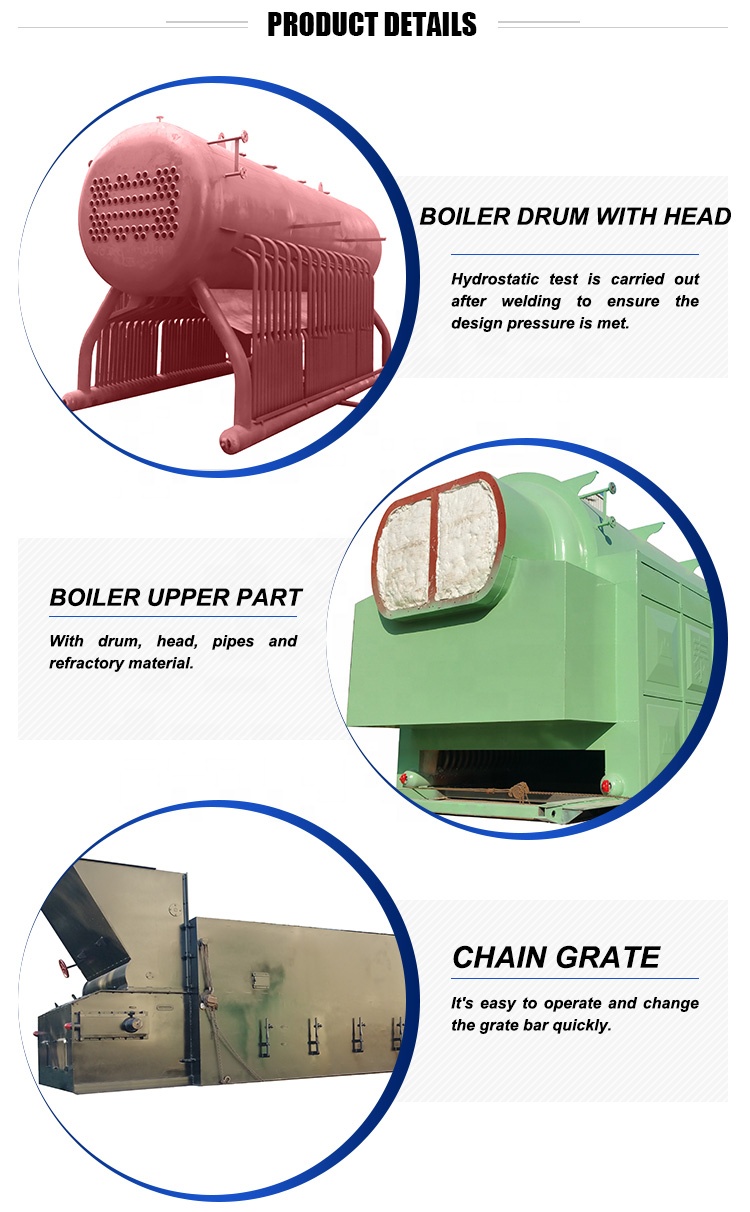
Why Is Controlling the Air-Fuel Ratio Essential for Efficient Combustion?
In every industrial boiler system—whether firing gas, oil, biomass, or coal—the combustion process hinges on how well the fuel is mixed with air. The air-fuel ratio determines whether the fuel burns completely, efficiently, and cleanly. Too much air dilutes the flame, reducing temperature and wasting heat. Too little air leads to incomplete combustion, creating soot, carbon monoxide (CO), and unburned fuel. Without precise air-fuel control, even the most advanced boiler systems experience efficiency loss, high emissions, and increased maintenance. That’s why maintaining the correct air-fuel ratio is essential to sustaining energy performance and meeting regulatory standards.
Controlling the air-fuel ratio is essential for efficient combustion because it ensures that fuel is burned completely with just the right amount of air, maximizing energy release and minimizing heat losses, CO emissions, and unburned fuel. Excess air lowers flame temperature and increases stack losses, while insufficient air causes incomplete combustion and pollutant formation. Precise control improves thermal efficiency, reduces fuel consumption, and ensures stable operation and emissions compliance.
The air-fuel ratio is not just a setting—it’s the foundation of combustion performance.
Controlling the air-fuel ratio ensures complete combustion and high boiler efficiency.True
Proper air-fuel mixing minimizes heat loss and pollutant formation, improving combustion stability and reducing operating costs.
🔍 Understanding the Air-Fuel Ratio and Combustion Zones
Term | Definition |
---|---|
Stoichiometric Ratio | Exact air amount needed to fully combust fuel (no excess air) |
Excess Air (%) | Additional air above stoichiometric to ensure complete burn |
Lean Burn | High air-fuel ratio, cooler flame, higher O₂, lower CO |
Rich Burn | Low air-fuel ratio, high CO, unburned fuel, soot |
→ Most industrial boilers operate with 10–25% excess air to ensure full combustion.
📊 Impact of Air-Fuel Ratio on Efficiency and Emissions
Condition | O₂ in Flue Gas (%) | CO (ppm) | Flame Temperature (°C) | Efficiency (%) |
---|---|---|---|---|
Too Little Air | <1.0 | >400 | 950–1050 | ↓ (unstable burn) |
Ideal Range | 2.5–4.5 | <100 | 1200–1400 | ✅ High |
Too Much Air | >6.0 | <50 | <1100 | ↓ (heat loss) |
→ Every 10% increase in excess air can reduce thermal efficiency by 0.5–1.0%.
🔧 How Excess Air Affects Boiler Performance
Excess Air Level | Effect on System |
---|---|
Low (<5%) | Incomplete combustion → CO, soot, instability |
Optimal (10–20%) | Full burnout, balanced flame, clean operation |
High (>25%) | Heat loss in stack gas, poor flame temp |
Indicator | Cause |
---|---|
High CO + Low O₂ | Too little air (rich burn) |
Low CO + High O₂ | Too much air (lean burn) |
Stable O₂ + Low CO | Ideal ratio, efficient combustion |
🛠 Instruments and Controls to Manage Air-Fuel Ratio
Tool | Role in Control |
---|---|
O₂ Analyzer | Measures excess air in flue gas |
CO/CO₂ Sensors | Detect incomplete combustion |
Flue Gas Temperature Probe | Indicates heat loss due to excess air |
Combustion Control System (CCS) | Automates air damper and fuel feed rate |
VFD for Fans | Modulates combustion air volume |
→ Advanced systems use oxygen trim and real-time feedback to keep the ratio optimized.
🧪 Case Study – 15 TPH Biomass Boiler
Initial Conditions:
Flue O₂ = 7.2%
CO = 140 ppm
Stack temp = 260°C
Efficiency = 78.1%
Action:
Installed O₂ trim controller
Adjusted fuel feeder and air flow balance
Results:
O₂ stabilized at 3.6%
CO dropped to <60 ppm
Efficiency rose to 84.6%
Monthly fuel savings: $11,000
📈 Ideal Excess Air Ranges by Fuel Type
Fuel Type | Recommended Excess Air (%) | O₂ in Flue Gas (%) |
---|---|---|
Natural Gas | 5–10% | 1–2.5% |
Fuel Oil | 10–15% | 2–4% |
Coal | 15–30% | 4–7% |
Biomass | 20–40% | 5–9% |
→ More variable fuels (biomass, coal) require higher excess air due to inconsistent combustion behavior.
🔍 Combustion Optimization Through Air-Fuel Control
Optimization Area | Benefit Achieved |
---|---|
Burner tuning | Balances air swirl and fuel pattern |
Fan modulation | Matches air supply to fuel load |
Load-based combustion control | Adapts air-fuel ratio to boiler load |
Oxygen trim | Reduces manual error and fluctuation |
Real-time monitoring | Ensures stable operation and alerts on faults |
→ Plants using intelligent air-fuel control systems report 3–10% fuel savings and more consistent compliance.
In conclusion, controlling the air-fuel ratio is critical to achieving efficient, complete, and clean combustion in industrial boilers. Too much or too little air leads to waste, emissions, and instability. With proper sensors, automation, and real-time tuning, boilers can consistently deliver optimal flame conditions, reduce excess fuel use, and remain well within emissions limits. Whether burning gas, coal, or biomass—combustion control begins with the right air-fuel balance.
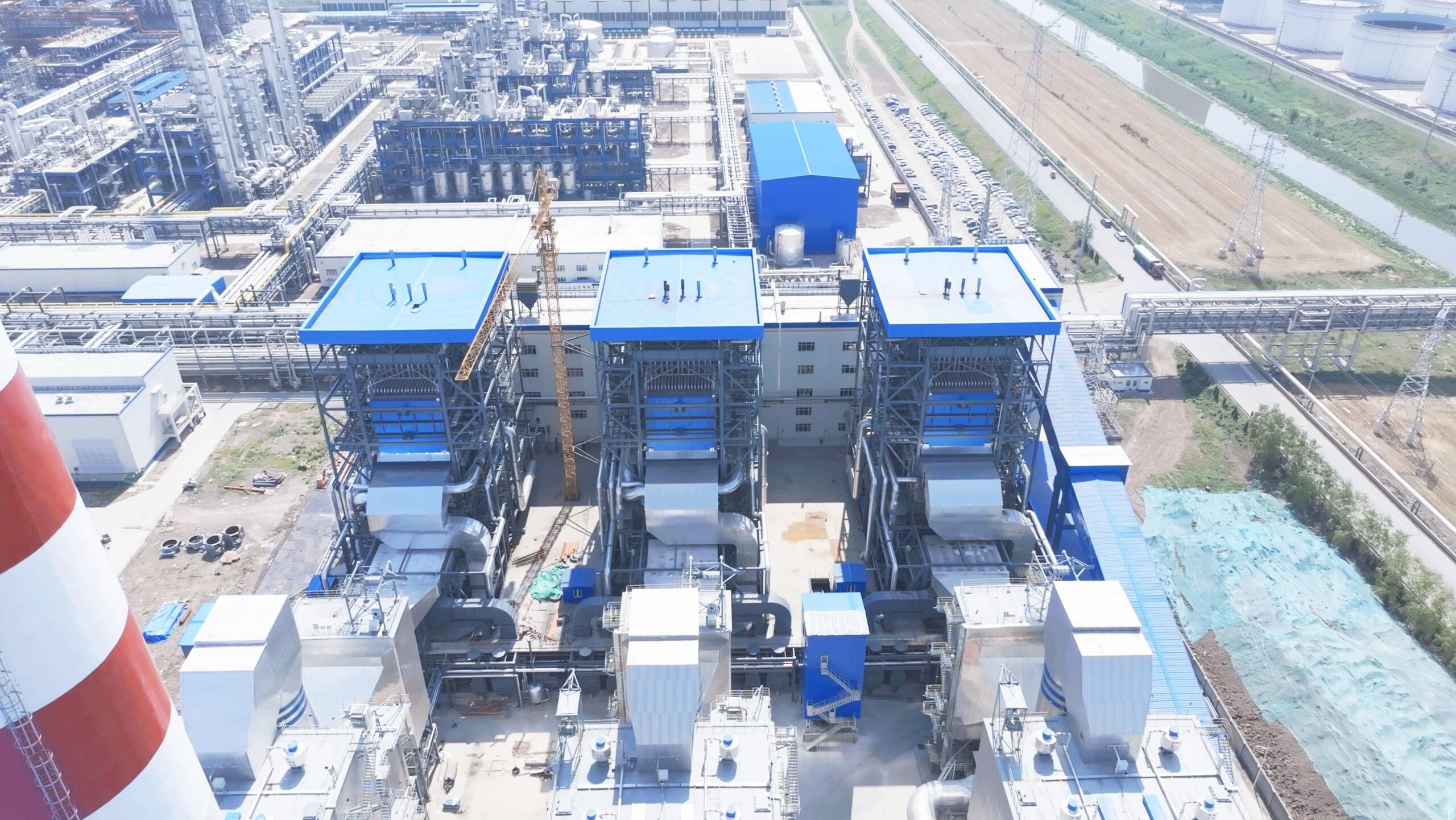
How Do Combustion Temperature and Furnace Design Influence Slagging and Efficiency?
In steam boiler systems—especially those using solid fuels like coal, biomass, or refuse-derived fuel (RDF)—slagging is a critical operational concern. Slagging refers to the formation of molten or semi-molten ash deposits on furnace walls and heat exchanger surfaces. It is heavily influenced by combustion temperature and furnace design. If temperatures are too high, ash components such as silica, iron, and alkali metals can fuse and adhere to surfaces, forming dense, insulating layers that obstruct heat transfer. At the same time, poor furnace design can promote flame impingement, hot spots, and poor gas flow, all of which exacerbate slag formation. These deposits lower heat transfer efficiency, cause unplanned shutdowns, and increase maintenance costs.
Combustion temperature and furnace design directly influence slagging and boiler efficiency by determining ash fusion behavior and how uniformly heat is distributed and absorbed. High temperatures above the ash fusion point cause slag to form on heat transfer surfaces, reducing efficiency and increasing wear. A well-designed furnace ensures even heat distribution, controlled flame temperature, and proper gas residence time to prevent slagging while maximizing energy absorption. Poor design or uncontrolled combustion leads to localized overheating, ash melting, and degraded performance.
Understanding this relationship is essential to maintain efficiency and avoid costly fouling and downtime.
Combustion temperature and furnace design have a direct impact on slagging behavior and boiler efficiency.True
High flame temperatures can cause ash to melt and stick to furnace walls, while poor furnace design may lead to uneven heating and slag accumulation.
🔥 Understanding Slagging and Its Formation Conditions
Term | Definition |
---|---|
Slagging | Melting and deposition of ash on furnace and heat exchanger walls |
Ash Fusion Temperature (AFT) | The temp at which ash components start to soften/melt |
Flame Temperature | Peak temperature in combustion zone (~1200–1600°C) |
Hot Gas Path | Route of flue gas from burner to boiler exit |
→ Slagging begins when local furnace temperatures exceed the AFT of ash compounds.
📏 Ash Fusion Temperature of Common Fuels
Fuel Type | Ash Fusion Temp (°C) | Slagging Risk at High Temp | Common Slagging Behavior |
---|---|---|---|
Bituminous Coal | 1250–1400 | Medium–High | Slag builds on side walls |
Lignite | 950–1200 | High | Low fusion → severe slagging |
Biomass (Straw) | 800–1050 | Very High | Alkali-silica slag at low temp |
Wood Chips | 1100–1250 | Moderate | Varies by bark, ash content |
Pet Coke | >1400 | Low–Moderate | Sulfur-based slag risk |
→ Combustion control must keep flame temperatures below AFT thresholds.
🛠 Furnace Design Parameters That Influence Slagging and Efficiency
Design Feature | Impact on Slagging | Impact on Efficiency |
---|---|---|
Furnace Volume | Larger volume allows flame burnout, less wall impingement | Promotes complete combustion |
Height-to-Width Ratio | Affects flame shape and gas residence time | Influences burnout and temperature profile |
Wall Water-Tube Arrangement | Reduces metal overheating and slag sticking | Enhances heat absorption surface |
Flame Direction and Angle | Poorly directed flames cause local slag zones | Controlled flame increases radiant efficiency |
Burner Positioning | Affects flame impingement and ash trajectory | Impacts mixing and combustion quality |
→ Proper geometry minimizes local hot spots and promotes even heat transfer.
📊 Effect of Temperature on Slag Formation and Efficiency
Flame Temperature (°C) | Slagging Severity | Heat Transfer Efficiency | Recommended Action |
---|---|---|---|
<1000 | Minimal | Lower (incomplete burn) | Increase combustion air preheat |
1100–1250 | Low–Moderate | High | Ideal range |
1300–1400 | High | Drops due to ash coating | Reduce burner intensity |
>1450 | Very High | Rapid decline | Re-design burner layout |
→ Every 1 mm of slag can reduce heat transfer by 5–10%.
🧪 Real-World Case Study – 25 TPH Lignite Boiler
Fuel: Lignite with 38% ash, AFT ~1050°C
Problem: Excessive slag buildup, tube fouling every 3 weeks
Root Cause:
Flame temperature measured >1350°C
Burner tilt directed flame at wall
Intervention:
Repositioned burner to widen flame spread
Added secondary air swirl to control flame shape
Adjusted excess air for flame cooling
Result:
Peak flame temp reduced to 1180°C
Slag intervals extended to 60 days
Efficiency improved by 6.4%
📈 Slagging vs. Efficiency – Performance Impact Table
Condition | Slag Formation | Thermal Efficiency (%) | Remarks |
---|---|---|---|
Optimized Furnace + 1150°C | Minimal | 86–88% | Best operating zone |
Hot Flame >1350°C | High | 78–82% | Slagging + fouling |
Poor Design + High Ash | Very High | <75% | Frequent shutdowns |
Low Flame <950°C | None | <80% | Incomplete combustion |
→ Temperature control and geometry design must be tuned together for peak output.
🔧 Strategies to Minimize Slagging and Improve Efficiency
Strategy | Benefit |
---|---|
Control Excess Air | Lowers flame temp, reduces slag risk |
Add Over-Fire Air (OFA) | Improves mixing, lowers NOx and slag |
Burner Angle Adjustment | Avoids flame impingement |
Use Additives (e.g., kaolin) | Raises ash fusion point in biomass |
Furnace Inspections & Deslagging | Restores heat transfer |
→ Combining operational and design improvements yields long-term gains.
In conclusion, combustion temperature and furnace design play a critical role in slagging behavior and overall boiler efficiency. High localized temperatures can cause ash to melt and stick to heat transfer surfaces, while poor furnace geometry intensifies these risks. By controlling flame characteristics and optimizing furnace layout, engineers can maintain cleaner surfaces, higher heat absorption, and lower maintenance frequency. In solid-fuel boilers especially, the fight against slagging begins at the heart of the flame.
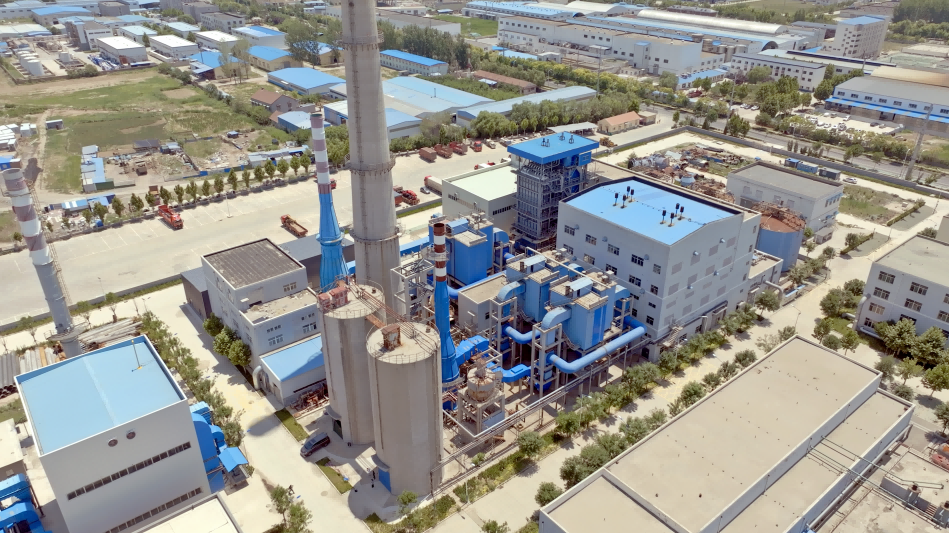
What Role Does Soot, Fouling, and Ash Buildup Play in Reducing Heat Transfer?
In any steam boiler system—particularly those burning solid fuels like coal, biomass, or waste—soot, fouling, and ash buildup are unavoidable operational by-products. Over time, these deposits accumulate on the fire-side (gas side) and water-side surfaces of heat exchangers, including the furnace walls, superheater, economizer, and air preheater. Even a thin layer of deposits acts as an insulator, blocking the transfer of heat from hot combustion gases to the water or steam inside the tubes. This increases flue gas temperature, reduces thermal efficiency, forces the boiler to consume more fuel for the same output, and accelerates wear and corrosion. Without regular maintenance and soot cleaning, boilers suffer efficiency losses of 5–15% or more.
Soot, fouling, and ash buildup reduce heat transfer efficiency by forming insulating layers on heat exchanger surfaces, impeding the flow of heat from flue gases to the working fluid. These deposits raise the thermal resistance of the heat transfer surface, causing increased flue gas temperatures, reduced steam generation, and higher fuel consumption. Over time, they can also lead to localized overheating, tube failure, and corrosion under deposits. Regular cleaning and preventive maintenance are essential to sustain efficient and safe boiler operation.
Clean surfaces mean more heat goes into your process—not up the stack.
Soot, fouling, and ash deposits insulate boiler heat exchange surfaces, reducing thermal efficiency.True
These deposits hinder effective heat transfer, leading to higher flue gas temperatures, reduced output, and increased fuel use.
🔍 What Are Soot, Fouling, and Ash in Boiler Systems?
Type | Source Material | Affects Surface | Typical Deposit Location |
---|---|---|---|
Soot | Unburned carbon particles | Furnace and superheater | Flame zone, water walls |
Fouling | Sticky ash, tar, or condensates | Tubes and walls | Superheater, economizer, APH |
Ash | Non-combustible minerals (SiO₂, Al₂O₃) | Fireside of heat exchangers | Tube banks, economizers, cyclones |
Scale | Water-side mineral deposits (e.g., CaCO₃) | Water-side tube surfaces | Steam drum, evaporator tubes |
→ All act as thermal insulators, lowering heat transfer and increasing fuel load.
📏 Measurable Impact of Deposits on Heat Transfer
Deposit Thickness | Type of Deposit | Heat Transfer Loss (%) | Efficiency Drop (%) |
---|---|---|---|
0.1 mm | Soot | ~5% | ~2–3% |
0.5 mm | Ash + soot | ~20% | ~5–7% |
1.0 mm | Hardened fouling | >30% | ~10–12% |
2.0 mm | Mixed ash/scale | >50% | ≥15% |
→ Even light fouling leads to significant thermal resistance and efficiency loss.
🔧 How Fouling Reduces Heat Transfer Efficiency
Effect | Description |
---|---|
Thermal Insulation | Deposits block heat from reaching tube metal |
Higher Flue Gas Temperature | More heat leaves with exhaust → stack losses ↑ |
Steam Output Reduction | Less heat input = less steam generation |
Increased Fuel Consumption | More fuel burned to compensate for lost energy |
Localized Overheating | Tube metal can overheat under deposits |
→ Long-term fouling also promotes creep, corrosion, and metal fatigue.
📊 Example – Biomass Boiler Performance With Fouling
Condition | Flue Gas Temp (°C) | Steam Output (TPH) | Efficiency (%) |
---|---|---|---|
Clean Tubes | 185 | 12.5 | 85.3 |
Moderate Fouling | 225 | 11.1 | 80.2 |
Severe Fouling | 270 | 10.0 | 74.8 |
→ Efficiency drop of 10.5% due to soot and ash buildup over 3 months.
🛠 Affected Boiler Areas and Cleaning Needs
Component | Typical Deposit Type | Maintenance Needed |
---|---|---|
Furnace Tubes | Soot, slag | Daily or weekly soot blowing |
Superheater | Ash, fouling | High-pressure soot blowers |
Economizer | Fly ash | Manual brushing or sonic cleaning |
Air Preheater (APH) | Sticky ash/tar | Offline cleaning with steam/air |
Water-Side Tubes | Scale (Ca, Mg, Fe) | Chemical descaling |
→ Neglected fouling shortens boiler life and increases unplanned downtime.
🧪 Case Study – Coal-Fired 35 TPH Boiler
Fuel: Bituminous coal with 24% ash
Problem: Stack temp increased from 220°C to 285°C
Findings:
Superheater and economizer tubes coated in 1.2 mm ash
PM emissions rose due to turbulence from fouling
Action:
Online soot blowing intensified
Offline hydroblasting during outage
Revised ash-handling cycle
Result:
Flue gas temp dropped to 195°C
Efficiency improved by 7.1%
Output restored to rated 35 TPH
📈 Cleaning Frequency vs. Performance Stability
Maintenance Frequency | Avg. Stack Temp (°C) | PM Emissions (mg/Nm³) | Steam Output Variability |
---|---|---|---|
Daily soot blowing | 190 | 32 | ±2% |
Weekly only | 240 | 68 | ±8% |
Bi-monthly | 270 | >90 | ±15% |
→ Regular maintenance = higher, more stable thermal efficiency.
🔧 Prevention and Monitoring Tools
Tool | Purpose |
---|---|
Infrared Thermography | Detects hot/cold spots → early fouling |
Flue Gas Temp Sensors | Rising values signal surface insulation |
Differential Pressure Gauges | Identify ash plugging in tubes |
Steam/Fuel Ratio Tracking | Declining values indicate heat loss |
Soot Blower Timers/PLC | Automate cleaning intervals |
→ Use data-driven diagnostics to avoid manual guesswork in fouling detection.
In conclusion, soot, fouling, and ash buildup play a major role in reducing heat transfer in industrial boilers. These deposits act like insulation on key heat transfer surfaces, dramatically lowering energy efficiency, increasing emissions, and raising operating costs. The solution is proactive—routine cleaning, smart monitoring, and effective fuel and air management. In heat transfer, cleanliness isn’t cosmetic—it’s performance-critical.
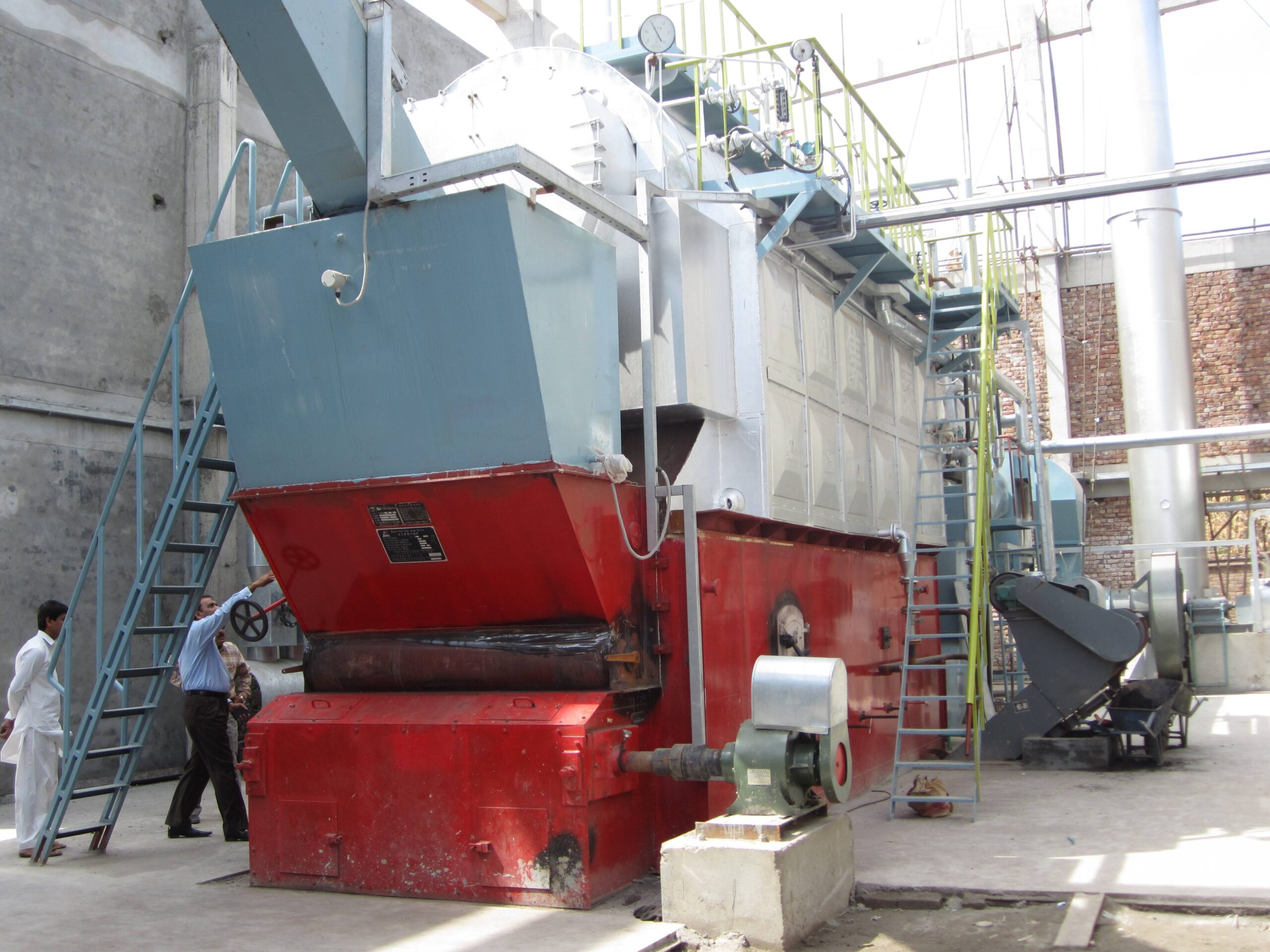
How Does Flue Gas Temperature Reflect Thermal Efficiency and Energy Losses?
In steam boiler systems, thermal efficiency is determined by how effectively combustion heat is transferred to water or steam—and how little is wasted. One of the most telling indicators of performance is flue gas temperature, the temperature of exhaust gases leaving the boiler through the stack. When flue gas temperature is high, it means that a significant portion of fuel energy wasn’t absorbed by the boiler’s heat exchangers and was instead lost to the atmosphere. If it’s too low, it risks condensation and corrosion. Monitoring and optimizing this parameter is essential to balance efficiency, safety, and emissions compliance.
Flue gas temperature reflects thermal efficiency and energy losses by indicating how much heat from combustion was transferred to the working fluid and how much was lost in the exhaust. Higher flue gas temperatures mean more unutilized energy, resulting in lower thermal efficiency and higher fuel consumption. Conversely, properly reduced flue gas temperatures (while staying above dew point) signify effective heat recovery and minimal energy loss. Monitoring this value helps operators detect fouling, poor combustion, or heat exchanger inefficiencies.
In other words, your stack temperature is a real-time efficiency gauge.
Flue gas temperature is a reliable indicator of boiler thermal efficiency and energy loss.True
Higher stack temperatures mean more energy is lost in exhaust gases, while lower, controlled flue temperatures indicate better heat recovery.
🔍 What Is Flue Gas Temperature and Why It Matters
Parameter | Description |
---|---|
Flue Gas Temperature | The exhaust gas temperature measured at the stack exit |
Thermal Efficiency | Ratio of heat absorbed by steam/water to fuel input |
Heat Losses | Unused energy escaping via flue gases or unburned fuel |
→ Typical stack temperatures range from 140°C to 300°C depending on fuel type and design.
📊 Flue Gas Temperature vs. Efficiency – Example Table
Flue Gas Temp (°C) | Approx. Thermal Efficiency (%) | Energy Loss (%) | Recommended Action |
---|---|---|---|
180 | 85–88 | Low | Optimal zone |
220 | 82–84 | Medium | Check soot or excess air |
260 | 78–81 | High | Clean surfaces, tune burners |
300 | <75 | Very High | Maintenance and inspection |
→ Every 20–30°C rise in flue gas temp can drop efficiency by 1–2%.
🔧 How Energy Is Lost Through the Flue Gas
Cause of High Flue Temp | Mechanism of Heat Loss |
---|---|
Soot and Ash Buildup | Insulates heat exchanger surfaces |
Excess Combustion Air | Cools flame and increases stack gas volume |
Poor Heat Transfer | Inefficient economizer or fouled tubes |
Low Water Flow | Reduces heat uptake on water side |
→ If not addressed, these losses accumulate into major fuel costs.
🛠 Calculating Energy Loss from Flue Gas Temperature
You don’t need a complex formula to estimate this. A simplified approach:
Rule of Thumb:
For every 22°C (40°F) rise above optimal flue gas temperature, efficiency drops by ~1%.
Example (Gas Boiler): |
---|
Design flue temp: 180°C |
Actual measured temp: 240°C |
Difference: 60°C → ~3% efficiency lost |
→ On a 10 TPH boiler, this could equal hundreds of tons of fuel wasted per year.
🧪 Case Study – 12 TPH Biomass Boiler
Initial Condition:
Flue gas temp = 275°C
Efficiency = 73.9%
Ash fouling in economizer
Corrective Measures:
Offline deslagging
Tuned excess air to reduce O₂ from 8% to 4.5%
Increased soot blower frequency
Result:
Flue gas temp reduced to 195°C
Efficiency improved to 83.4%
Fuel savings: 580 tons/year
📈 Optimal Flue Gas Temperature Ranges by Fuel Type
Fuel Type | Target Stack Temp (°C) | Dew Point Risk Zone (°C) | Remarks |
---|---|---|---|
Natural Gas | 140–180 | ~55–65 | Risk of condensation below 130°C |
Fuel Oil | 180–230 | ~90–110 | Keep above acid dew point |
Coal | 200–260 | ~120–140 | Balance slagging and corrosion |
Biomass | 200–250 | ~100–130 | Varies by moisture and ash type |
→ Always stay above the acid dew point to prevent sulfuric acid corrosion.
🔍 Signs That Flue Gas Temperature Indicates a Problem
Symptom | Possible Cause |
---|---|
Rising flue temp | Fouling, slagging, excess air |
Stable but high temp | Under-designed heat exchangers |
Fluctuating flue temp | Load swings, unstable combustion |
Sudden spike | Soot blower failure, air leak, fouled tubes |
→ Regular monitoring allows predictive maintenance and tuning.
🛠 Best Practices to Optimize Flue Gas Temperature
Practice | Impact |
---|---|
Regular Soot Blowing | Removes ash insulation |
Economizer Installation | Recovers heat to preheat feedwater |
O₂ Trim Control System | Maintains ideal air-fuel ratio |
Condensing Heat Recovery (Gas) | Pushes efficiency above 90% |
Combustion Tuning | Maximizes energy absorption |
→ Integrated combustion and maintenance control is key to thermal efficiency.
In conclusion, flue gas temperature is a powerful and immediate indicator of boiler thermal efficiency and energy losses. A rising stack temperature signals wasted fuel, poor heat exchange, or combustion issues. By monitoring and optimizing flue gas temperature through cleaning, tuning, and proper system design, operators can recover lost energy, lower emissions, and maintain peak boiler performance. In short: lower stack temps (within safe limits) = higher profits and cleaner operation.
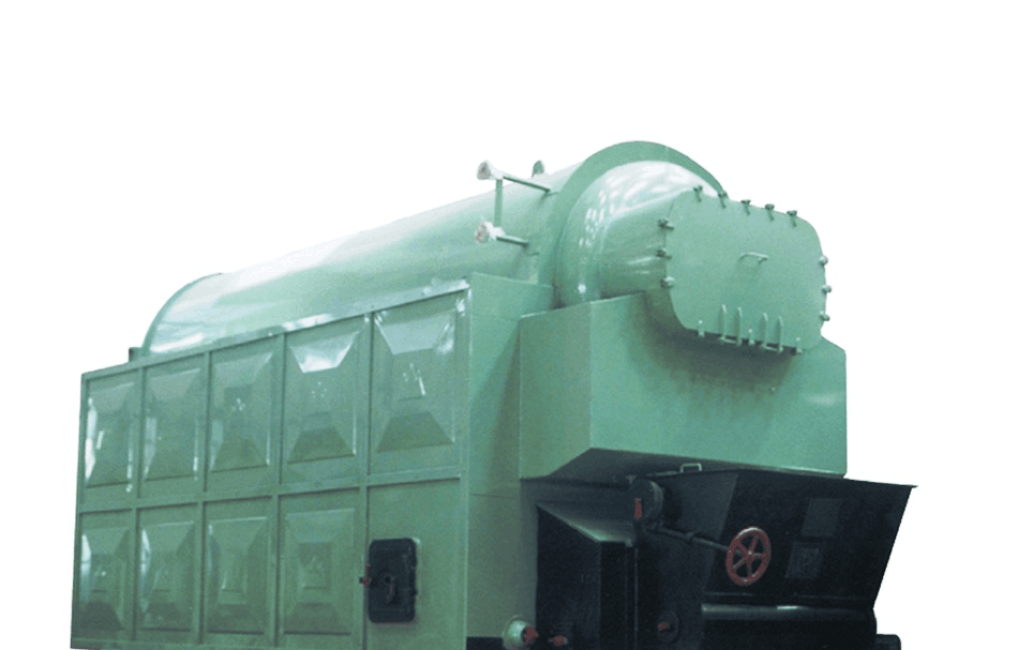
What Operational and Maintenance Practices Are Key to Sustaining High Efficiency?
Industrial boiler systems are high-energy equipment designed to convert fuel into steam with maximum heat transfer and minimum waste. However, over time, efficiency deteriorates due to fouling, poor combustion, air leaks, and component wear. Without a structured approach to operations and maintenance (O&M), boilers suffer from high flue gas temperatures, incomplete combustion, unplanned shutdowns, and rising emissions. To ensure long-term efficiency, safety, and regulatory compliance, operators must implement a disciplined set of best practices that proactively manage combustion quality, surface cleanliness, water treatment, and real-time monitoring.
Sustaining high boiler efficiency requires a combination of operational practices such as optimal air-fuel ratio control, load balancing, and flue gas monitoring, along with maintenance activities like regular soot blowing, descaling, burner inspection, and water treatment. These practices ensure clean heat exchange surfaces, stable combustion, and minimal energy loss. Preventive and predictive maintenance, guided by performance data, allows early detection of inefficiencies and helps avoid costly downtime.
A high-efficiency boiler is not born—it is maintained.
Operational and maintenance practices are critical to maintaining high thermal efficiency in steam boilers.True
Practices like burner tuning, flue gas monitoring, and surface cleaning prevent energy losses and support stable, clean combustion.
🔍 Essential Operational Practices for Sustaining Efficiency
Practice | Efficiency Benefit |
---|---|
Air-Fuel Ratio Tuning | Ensures complete combustion and minimizes CO/NOx |
Oxygen Trim Control | Maintains optimal excess air throughout load cycles |
Steam Load Management | Prevents cycling losses and maintains stable firing |
Boiler Load Matching | Operate at or near peak efficiency zone (60–85%) |
Flue Gas Monitoring | Detects fouling, improper combustion, or air leaks |
Condensate Recovery | Saves heat and water, reduces fuel use |
→ These practices are continuous—not periodic—and require automation and trained operators.
🛠 Maintenance Practices That Protect Efficiency
Maintenance Activity | Interval | Efficiency Impact |
---|---|---|
Soot Blowing (manual/auto) | Daily/weekly | Prevents insulation of heat transfer surfaces |
Tube Cleaning (offline) | Monthly/quarterly | Removes hardened ash or slag |
Burner Inspection & Tuning | Monthly | Ensures proper flame shape and fuel delivery |
Feedwater Treatment Monitoring | Daily | Prevents scale buildup on water-side surfaces |
Descaling (acid or mechanical) | Biannually | Restores water-side heat transfer efficiency |
Gasket and Seal Checks | Monthly | Prevents air ingress and O₂ dilution |
→ A clean boiler is an efficient boiler—maintenance protects every kcal of fuel input.
📏 Real-World Example: Impact of Sustained O&M on Performance
Boiler Type | Without Maintenance | With Optimized O&M |
---|---|---|
Flue Gas Temp (°C) | 270 | 190 |
Steam Output (TPH) | 8.6 | 10.2 |
Thermal Efficiency (%) | 74.3 | 85.1 |
Fuel Consumption (kg/hr) | 2,950 | 2,400 |
→ Efficiency gain of ~11%, fuel savings of 550 kg/hr, and stable operation achieved.
📊 Efficiency Monitoring and Diagnostic Tools
Tool | What It Detects |
---|---|
O₂ and CO Analyzers | Air-fuel balance and combustion completeness |
Flue Gas Temp Sensors | Heat transfer efficiency, soot fouling |
Differential Pressure Gauges | Economizer blockage, air-side fouling |
Steam-to-Fuel Ratio Logger | Real-time energy performance indicator |
Thermal Imaging Camera | Hot spots, refractory damage, slag deposits |
→ These tools enable predictive maintenance based on actual performance—not calendar guesses.
🧪 Case Study – Gas-Fired Boiler in Food Processing
Initial Condition:
Fuel cost = $85,000/month
Flue gas temp = 245°C
Frequent CO spikes, high O₂ (7%)
O&M Improvements:
Installed O₂ trim system
Burner retuned and reoriented
Condensate recovery increased from 58% to 88%
Implemented monthly offline tube cleaning
Result:
Flue gas temp dropped to 178°C
O₂ stabilized at 3.5%, CO <50 ppm
Fuel savings = $13,200/month
Thermal efficiency improved by 9.3%
🧰 O&M Action Checklist for High Efficiency
Area | Task | Frequency |
---|---|---|
Combustion | Burner tuning, air damper control | Monthly / load change |
Heat Transfer Surfaces | Soot blowing, tube cleaning | Daily to quarterly |
Water Treatment | Conductivity, pH, hardness tests | Daily |
Instrumentation | Calibrate sensors (O₂, CO, flow) | Monthly |
Flue Gas Analysis | NOx, CO, O₂, stack temp logging | Continuous / weekly |
Condensate System | Return ratio check | Weekly |
→ A routine calendar and logging system ensures practices aren’t skipped or delayed.
📈 Efficiency Losses When O&M Is Neglected
Problem | Efficiency Loss (%) | Root Cause |
---|---|---|
Fouled tubes (1 mm soot) | 7–10% | Missed soot blowing |
Scaling on water-side | 5–15% | Inadequate feedwater control |
Incorrect air-fuel ratio | 3–8% | Lack of burner tuning or sensor drift |
Unrecovered condensate | 5–10% | Return system failure |
→ Inefficiencies stack up quickly without prevention.
🔍 Integrating O&M with Efficiency KPIs
KPI | Optimal Value | O&M Link |
---|---|---|
Steam-to-Fuel Ratio | ≥11 kg/kg (gas) | Indicates fuel conversion efficiency |
Flue Gas Temp | 170–190°C (gas) | Should stay steady if surfaces are clean |
CO (ppm) | <100 | High values = poor combustion |
O₂ (%) | 2.5–4.5% | Excess air tuning |
Blowdown Rate (%) | 2–5% | Proper water quality management |
→ Track these indicators weekly or via automation.
In conclusion, sustaining high efficiency in boiler systems is only possible through a disciplined blend of operational excellence and proactive maintenance. By focusing on combustion control, cleanliness, monitoring, and preventive action, operators can ensure that every unit of fuel is used to its full potential. Efficiency is not a fixed number—it’s a function of how well your team manages the system every day. In high-performance boiler operations, efficiency is engineered—and preserved.
🔍 Conclusion
Optimizing heat transfer and combustion efficiency in coal-fired boilers requires a holistic understanding of fuel behavior, airflow management, system design, and maintenance practices. Regular monitoring of ash content, flame stability, and heat exchanger cleanliness is vital for preventing energy losses and ensuring compliance with environmental standards. When these factors are well-managed, industrial coal-fired boilers can deliver stable, efficient, and cost-effective performance over the long term.
📞 Contact Us
💡 Want to improve your coal boiler’s efficiency and reduce fuel costs? Our engineers specialize in combustion optimization, heat transfer diagnostics, and operational training tailored to coal-fired systems.
🔹 Contact us today and unlock the full efficiency potential of your coal-fired boiler! 🏭🔥📊
FAQ
What factors influence heat transfer efficiency in coal-fired boilers?
Several factors impact heat transfer, including:
Boiler tube design and surface area
Soot and ash deposition on heat exchange surfaces
Flame temperature and residence time
Water and steam flow rates
Clean, properly maintained tubes and optimal combustion conditions are essential for efficient heat exchange from flue gases to steam.
How does coal quality affect combustion efficiency?
Coal with high ash content, moisture, or variable calorific value reduces combustion efficiency. Low-quality coal results in incomplete combustion, more unburned carbon, and higher emissions. Consistent and well-prepared coal enhances boiler stability and energy output.
Why is air-to-fuel ratio important for combustion efficiency?
An ideal air-to-fuel ratio ensures complete combustion. Too much excess air leads to heat loss through flue gases; too little air causes incomplete combustion and high CO/soot levels. Proper oxygen control is vital for both efficiency and emissions compliance.
What role does ash behavior play in heat transfer and performance?
Ash with a low fusion point may slag and foul boiler surfaces, forming insulating layers that reduce heat transfer and increase fuel consumption. Effective soot-blowing and ash management systems are critical in coal-fired boiler operations.
How can combustion and heat transfer efficiency be improved in coal boilers?
Use of pulverized or graded coal for better combustion
Installation of low-NOx burners and O₂ trim systems
Regular cleaning of heat exchanger surfaces
Combustion tuning using flue gas analyzers
Use of additives to reduce slagging and fouling
These practices enhance overall thermal performance, reduce downtime, and improve emissions control.
References
Coal-Fired Boiler Efficiency Factors – https://www.energy.gov
Combustion Optimization in Coal Boilers – https://www.sciencedirect.com
Ash Behavior and Boiler Fouling – https://www.researchgate.net
Air-Fuel Ratio Management in Solid Fuel Boilers – https://www.epa.gov
Fuel Preparation and Handling for Efficiency – https://www.bioenergyconsult.com
Heat Exchanger Surface Maintenance – https://www.mdpi.com
Boiler Tube Fouling and Cleaning Techniques – https://www.iea.org
Coal Quality and Combustion Control – https://www.energysavingtrust.org.uk
Advanced Coal Boiler Control Systems – https://www.automation.com
Thermal Performance in Industrial Coal Systems – https://www.asme.org
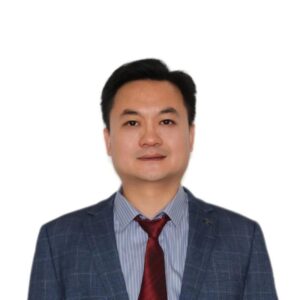