How to Optimize Industrial Gas-Fired Boiler Performance for Lower Operating Costs
Industrial gas-fired boilers are widely used for their high thermal efficiency, cleaner combustion, and ease of control. But even the most advanced systems can become inefficient and costly without proper performance optimization. Poor combustion control, heat losses, and inconsistent maintenance can lead to excessive fuel consumption, equipment degradation, and unnecessary operating expenses. Optimizing your gas-fired boiler ensures maximum return on investment and long-term operational stability.
To optimize industrial gas-fired boiler performance for lower operating costs, focus on combustion tuning, proper air-to-fuel ratio control, flue gas heat recovery, regular maintenance of heat exchange surfaces, and integration of smart monitoring systems. Efficiency improvements can be achieved by reducing excess air, maintaining clean burners and economizers, and adjusting load management strategies. Incorporating real-time analytics and preventive diagnostics can help minimize downtime, extend equipment life, and reduce fuel waste.
Let’s break down the key strategies for driving better efficiency and lowering your boiler’s operating costs.
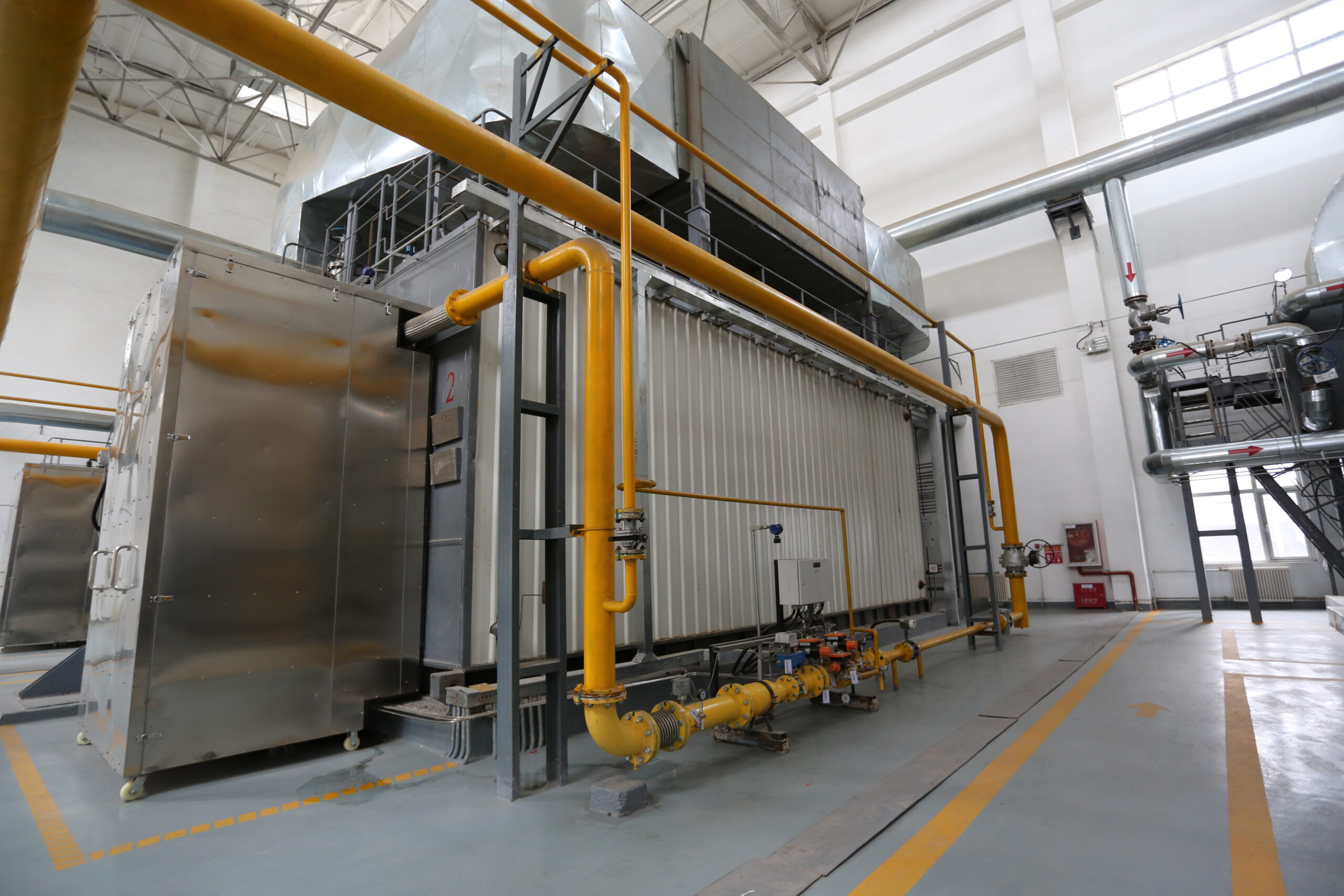
How Does Burner Tuning and Air-Fuel Ratio Optimization Reduce Fuel Consumption?
In industrial boilers, fuel costs can account for over 70% of total operating expenses. Any deviation in combustion balance—even by a few percentage points—can lead to significant energy losses and excess emissions. One of the most effective ways to minimize fuel consumption without compromising performance is through precise burner tuning and air-fuel ratio optimization. Burners that are out of alignment, clogged, or poorly adjusted waste fuel through incomplete combustion or excessive excess air. When air-fuel control is calibrated properly, combustion becomes more efficient, releasing maximum energy per unit of fuel and reducing wasteful heat losses.
Burner tuning and air-fuel ratio optimization reduce fuel consumption by ensuring that fuel burns completely and efficiently with just the right amount of air. Proper tuning aligns burner settings, damper positions, and flame patterns to maximize heat release while minimizing unburned fuel and excess air. Too little air causes incomplete combustion and CO emissions, while too much air cools the flame and increases stack losses. Optimizing the air-fuel ratio improves flame stability, lowers flue gas temperature, and increases heat transfer efficiency—leading to measurable fuel savings.
In boiler combustion, precision isn’t optional—it’s profitable.
Optimized air-fuel ratio through burner tuning reduces fuel consumption by maximizing combustion efficiency.True
Precise control avoids incomplete combustion and minimizes heat losses due to excess air.
🔍 What Is Burner Tuning?
Tuning Activity | Purpose |
---|---|
Burner Alignment | Ensures uniform fuel distribution across flame |
Damper Adjustment | Controls primary and secondary air mixing |
Fuel Nozzle Inspection/Cleaning | Maintains proper spray and atomization |
Ignition and Flame Stability Checks | Prevents flameout, CO spikes |
Control Loop Calibration | Ensures sensors (O₂, temp, CO) provide accurate feedback |
→ Tuning is about matching combustion conditions to design performance.
📏 Understanding Air-Fuel Ratio Optimization
Term | Description |
---|---|
Stoichiometric Ratio | Exact air required for complete combustion of the fuel |
Excess Air | Air supplied above stoichiometric level to ensure full burnout |
O₂ in Flue Gas (%) | Key indicator of excess air—optimal range: 3–5% for most fuels |
Condition | O₂ (%) | CO (ppm) | Fuel Use Impact |
---|---|---|---|
Too Little Air | <2 | >300 | Incomplete burn → waste ↑ |
Optimal Air-Fuel Ratio | 3–4.5 | <100 | ✅ Maximum efficiency |
Too Much Air | >6 | <50 | Stack losses ↑ → fuel ↑ |
→ Air-fuel optimization is about finding and maintaining that sweet spot.
📊 Impact of Air-Fuel Ratio on Fuel Consumption
O₂ in Flue Gas (%) | Flue Gas Temp (°C) | Efficiency (%) | Fuel Use (kg/hr) | Fuel Savings Potential |
---|---|---|---|---|
7.0 | 255 | 78.5 | 6,500 | – |
4.5 | 195 | 86.3 | 5,800 | ✅ ~700 kg/hr saved |
2.0 | 180 | 83.0 | 5,900 | ⚠ CO ↑ (incomplete burn) |
→ Proper tuning saves fuel while maintaining safe and stable combustion.
🧪 Case Study – Gas-Fired Boiler Burner Tuning
System: 20 TPH natural gas boiler
Initial Readings:
O₂ = 6.8%, Flue gas temp = 240°C, CO = 45 ppm
Thermal efficiency = 80.1%, Fuel usage = 4,200 Nm³/hr
Action:
Recalibrated O₂ sensors
Adjusted air dampers to reduce excess air
Retuned burner flame shape and mixing
Post-Tuning Results:
O₂ = 3.9%, Flue gas temp = 185°C
Efficiency = 88.2%, Fuel usage = 3,750 Nm³/hr
Fuel savings = ~450 Nm³/hr (~10.7% reduction)
→ Better combustion = less gas burned per ton of steam.
🔧 Tools and Instruments for Tuning and Optimization
Instrument / System | Function |
---|---|
Flue Gas Analyzer (O₂, CO, NOx) | Measures combustion quality |
Thermocouples / RTDs | Monitor flame and flue gas temperatures |
SCADA with Real-Time Tuning Dashboard | Tracks efficiency, recommends adjustments |
Damper Position Indicators | Visualize air flow changes |
Auto O₂ Trim Control System | Continuously adjusts excess air automatically |
→ Tuning is most effective when paired with real-time combustion feedback.
🛠 Best Practices for Burner Tuning and Air-Fuel Control
Practice | Benefit |
---|---|
Schedule seasonal tuning | Accounts for temperature/humidity impact |
Clean nozzles and diffusers regularly | Maintains consistent fuel spray and mixing |
Log and trend O₂/CO/efficiency data | Identifies drift or degradation |
Calibrate sensors quarterly | Prevents errors in air-fuel feedback loops |
Perform burner visual inspections | Detects flame instability and maldistribution |
→ Fuel economy is not just in the fuel—it’s in the settings and sensors.
📈 Annual Fuel Savings from Burner Optimization
Boiler Size (TPH) | Fuel Type | Pre-Tuning Efficiency | Post-Tuning | Annual Savings |
---|---|---|---|---|
15 TPH | Biomass | 72.4% | 82.0% | ~1,200 tons |
20 TPH | Natural Gas | 79.6% | 88.1% | ~95,000 Nm³ |
40 TPH | Coal | 76.2% | 85.3% | ~4,500 tons |
→ Burner tuning is often the fastest ROI in boiler optimization.
In conclusion, burner tuning and air-fuel ratio optimization are cornerstone practices for reducing fuel consumption and maximizing boiler efficiency. By ensuring that combustion is complete and air is supplied in just the right amount, operators can reduce fuel waste, cut emissions, and extend equipment life. These optimizations don’t require massive capital—but they deliver massive impact. In thermal systems, the best savings come not from burning harder—but burning smarter.
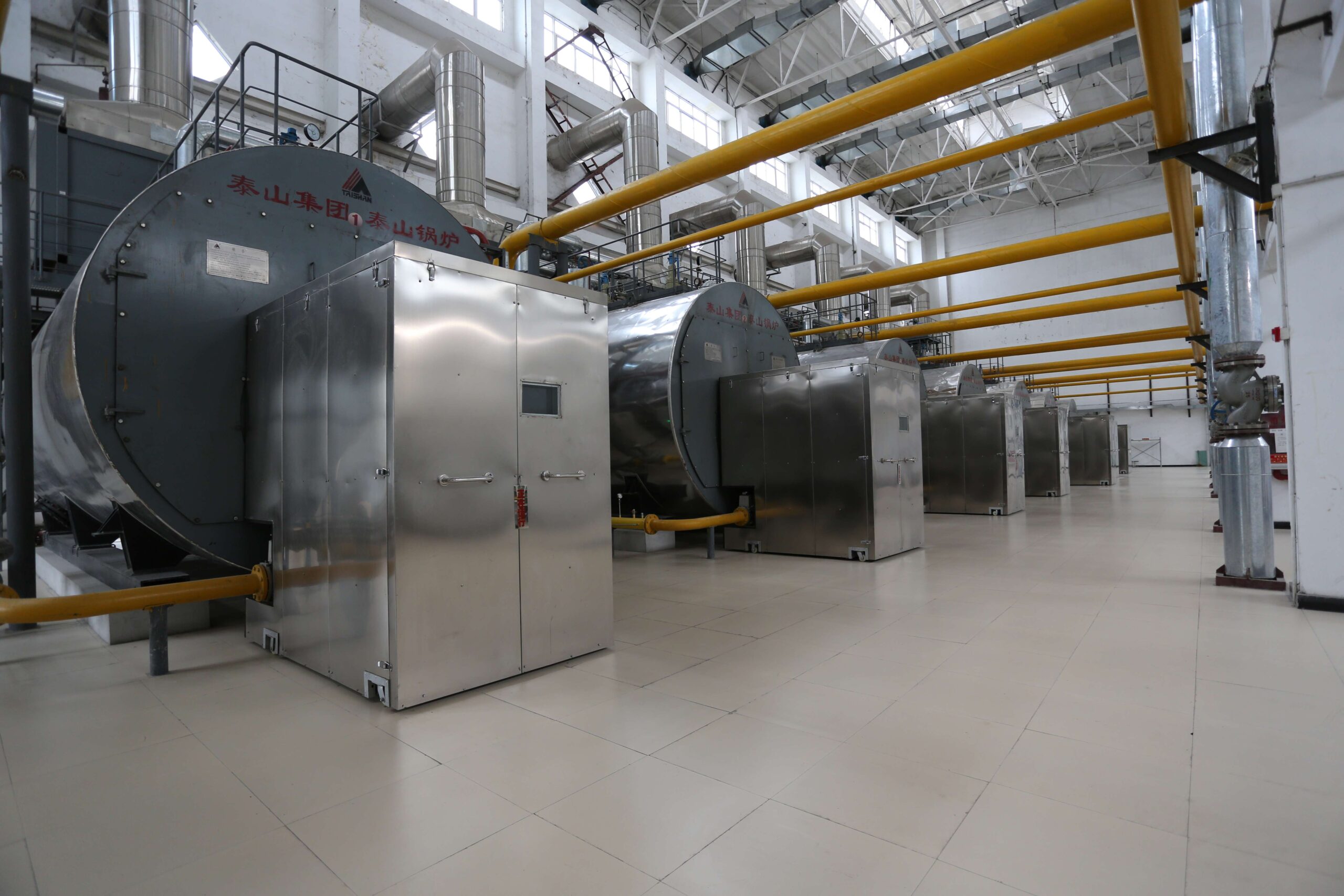
Why Is Flue Gas Heat Recovery Critical for Maximizing Thermal Efficiency?
In every boiler system—regardless of fuel type—a significant amount of heat escapes through the flue gas. Even in well-tuned combustion systems, stack temperatures can exceed 180–250°C. This heat represents unrecovered energy that was paid for but never used. The higher the flue gas temperature, the greater the potential for energy loss—and the larger the opportunity for savings. Flue gas heat recovery systems like economizers, air preheaters, and condensing heat exchangers capture this otherwise wasted energy and redirect it back into the system. This process dramatically improves thermal efficiency, reduces fuel consumption, and lowers emissions.
Flue gas heat recovery is critical for maximizing thermal efficiency because it captures residual heat from exhaust gases and transfers it to feedwater, combustion air, or process fluids. This reduces the energy needed to raise steam, preheat air, or warm water, thereby decreasing fuel use and increasing boiler efficiency. Without recovery, this heat escapes through the stack, resulting in higher operating costs and lost energy. Installing economizers or condensing systems can improve thermal efficiency by 5–15%, making it one of the most impactful efficiency upgrades in any boiler system.
In a boiler, flue gas is not just waste—it’s a second chance to save energy.
Flue gas heat recovery significantly boosts thermal efficiency by reclaiming heat that would otherwise be lost through the stack.True
This recovered energy reduces the fuel needed to heat feedwater or combustion air, improving overall system efficiency.
🔍 How Flue Gas Heat Recovery Works
Component | Function |
---|---|
Economizer | Transfers flue gas heat to incoming feedwater |
Air Preheater (APH) | Preheats combustion air using exhaust heat |
Condensing Heat Exchanger | Captures latent heat from water vapor in flue gas |
Heat Pipe / Recuperator | Transfers heat to process streams or district heating |
→ These systems lower flue gas temperature and redirect energy back into the cycle.
📏 Typical Flue Gas Temperatures and Recovery Potential
System Type | Exit Flue Gas Temp (°C) | Recovery Method | Estimated Efficiency Gain (%) |
---|---|---|---|
Standard Boiler | 220–260 | Economizer | 4–7 |
CFB or Biomass Boiler | 180–220 | Economizer + APH | 6–9 |
Gas-Fired Condensing | 60–90 | Condensing heat exchanger | 8–15 |
Coal-Fired Utility | 150–200 | Economizer + APH + Sootblowers | 5–10 |
→ Every 10°C reduction in flue gas temperature recovers up to 1% of fuel energy.
📊 Example: Heat Recovery from Flue Gas
Parameter | Before Recovery | After Recovery |
---|---|---|
Flue Gas Temperature (°C) | 245 | 170 |
Feedwater Temp (°C) | 90 | 140 |
Boiler Efficiency (%) | 79.5 | 87.2 |
Fuel Consumption (kg/hr) | 6,500 | 5,720 |
Efficiency Gain | – | +7.7% |
→ Economizer installation reduced flue gas temperature by 75°C and saved 780 kg/hr of fuel.
🧪 Case Study – Biomass Boiler with Economizer Upgrade
System: 15 TPH biomass boiler, stack temp 230°C
Intervention: Installed finned-tube economizer on flue duct
Result:
Flue temp reduced to 155°C
Feedwater temp raised from 85°C to 132°C
Efficiency improved from 74.2% to 82.9%
Annual fuel savings: ~1,100 tons
→ ROI achieved in less than 18 months.
📈 Benefits of Flue Gas Heat Recovery
Benefit | Explanation |
---|---|
Increased Boiler Efficiency | More energy extracted from the same fuel |
Reduced Fuel Consumption | Less fuel needed to reach desired steam temp |
Lower CO₂ and NOx Emissions | Lower combustion volume reduces emissions per MWh |
Improved Steam Output Stability | Preheated water leads to smoother operation |
Reduced Boiler Load | System runs closer to design setpoint |
→ Heat recovery transforms efficiency from a theoretical concept into real savings.
🔧 Key Factors in Heat Recovery Design
Factor | Why It Matters |
---|---|
Flue Gas Composition | Moisture and acid gases affect corrosion risk |
Soot and Fouling Behavior | Impacts cleaning frequency and recovery consistency |
Material Selection | Stainless steel or alloy for acidic condensates |
Maintenance Access | Easy access for soot blowing and tube inspection |
Space Constraints | Determines whether economizer or condensing system |
→ Engineering heat recovery systems requires balance between efficiency and durability.
🛠 Maintenance and Monitoring for Recovery Systems
Task | Frequency | Benefit |
---|---|---|
Tube Cleaning (Sootblowing) | Weekly to monthly | Prevents insulation of surfaces |
Flue Gas Temp Logging | Continuous | Tracks performance drop-off |
Corrosion Inspection | Quarterly | Avoids tube failure in condensing zones |
Drain System Check (Condensers) | Monthly | Ensures water and acid are safely removed |
Water Quality Monitoring | Ongoing | Prevents scaling on feedwater side |
→ Even efficient recovery systems require care to stay efficient.
In conclusion, flue gas heat recovery is one of the most effective and accessible ways to enhance thermal efficiency in industrial boiler systems. By reclaiming energy that would otherwise be lost, it reduces fuel consumption, lowers emissions, and improves system performance. Whether through an economizer, air preheater, or condensing exchanger, heat recovery transforms exhaust into value. In thermal systems, what you recover is just as important as what you burn.

What Role Does Regular Maintenance Play in Maintaining High-Performance Operation?
Industrial boilers, power plant systems, and heat exchangers are designed for high performance—but over time, performance inevitably degrades without regular maintenance. Fouling, scale buildup, drift in sensors, air leaks, and mechanical wear all contribute to declining efficiency, rising emissions, and even dangerous operating conditions. Regular maintenance isn’t just about fixing what’s broken—it’s about preserving the finely balanced conditions needed for efficient combustion, heat transfer, and system integrity. Neglecting this leads to energy loss, equipment failure, and increased operating costs. Proactive maintenance is therefore essential to ensure safe, efficient, and reliable performance across the system’s lifespan.
Regular maintenance plays a critical role in sustaining high-performance operation by preventing the accumulation of efficiency losses, ensuring optimal heat transfer, maintaining accurate sensor readings, and preserving mechanical integrity. Routine tasks such as cleaning heat exchanger surfaces, calibrating instruments, checking seals, and tuning combustion systems ensure the boiler operates at peak efficiency, consumes less fuel, and emits fewer pollutants. Without maintenance, even the best-designed systems experience rapid degradation in output and reliability.
Maintenance is not a cost—it’s a strategic investment in continuous performance.
Regular maintenance is essential for sustaining high-performance operation in boilers and thermal systems.True
It prevents gradual degradation from fouling, drift, wear, and corrosion, all of which reduce efficiency and reliability.
🔍 Why Maintenance Is a Pillar of Performance
Maintenance Objective | Efficiency Benefit |
---|---|
Remove Fouling from Surfaces | Restores heat transfer → reduces fuel usage |
Calibrate Sensors | Ensures correct combustion and steam control |
Inspect and Clean Burners | Maintains flame stability and complete combustion |
Check for Steam and Air Leaks | Prevents invisible heat and pressure loss |
Service Pumps and Valves | Ensures stable feedwater and fuel delivery |
→ Each task helps maintain the fine balance of thermal efficiency.
📏 Key Performance Areas Affected by Maintenance
System Component | Maintenance Action | Performance Risk if Ignored |
---|---|---|
Economizer / Superheater | Soot blowing and cleaning | High flue gas temp, low steam output |
Burner System | Inspection, tuning, alignment | CO emissions, fuel waste, unstable flame |
O₂ / CO Analyzers | Sensor calibration | Incorrect air-fuel ratio, efficiency ↓ |
Feedwater System | Descaling, valve checks | Boiler scaling, pressure control issues |
Insulation & Seals | Inspection and repair | Radiation loss, temperature instability |
→ A single overlooked issue can degrade multiple efficiency parameters.
📊 Efficiency Loss Trends Without Maintenance
Maintenance Lapse | Typical Time to Degradation | Efficiency Loss (%) |
---|---|---|
Soot Accumulation | 1–3 weeks | 3–10 |
Sensor Drift (O₂) | 1–2 months | 2–5 |
Scaling in Water Side | 2–6 months | 1–4 |
Leaking Steam Traps | 3–6 months | 2–8 |
Air Duct Leaks | Gradual | 1–6 |
→ Small issues accumulate quickly without scheduled intervention.
🧪 Case Study – Maintenance-Driven Efficiency Recovery
System: 25 TPH coal-fired boiler
Problem: Thermal efficiency dropped from 84% to 76% over six months
Findings:
Economizer fouled (flue gas temp increased by 40°C)
O₂ sensor was off by 1.2% (reading too low)
Air leaks around burner assembly
Maintenance Action:
Cleaned economizer tubes
Recalibrated sensors and checked dampers
Sealed air leaks and retuned burners
Result:
Efficiency restored to 85.1%
Fuel savings of ~1,600 tons/year
ROI on maintenance costs achieved in 4 months
→ Maintenance turned a declining system back into a high-performance unit.
🔧 Recommended Maintenance Schedule for High Efficiency
Task | Frequency | Benefit |
---|---|---|
Soot Blowing / Tube Cleaning | Daily to weekly | Keeps heat surfaces clean |
Burner Inspection and Tuning | Monthly to quarterly | Maintains combustion quality |
Sensor Calibration (O₂, CO, Temp) | Monthly | Enables accurate air-fuel control |
Leak Detection and Repair | Monthly | Prevents energy and pressure loss |
Water Treatment System Check | Weekly | Prevents scale and corrosion |
Boiler Blowdown Management | Daily or automated | Controls TDS, improves heat exchange |
→ Following a schedule ensures issues are resolved before they cost energy.
📈 Benefits of Regular Maintenance for Performance & Cost
Benefit | How Maintenance Delivers It |
---|---|
Higher Thermal Efficiency | Clean surfaces, tuned combustion, accurate sensors |
Lower Fuel Consumption | Reduced stack loss, better heat recovery |
Stable Steam Output | Prevents interruptions from scaling, fouling |
Longer Equipment Lifespan | Avoids stress from hot spots, corrosion |
Reduced Downtime and Outages | Catches failures before escalation |
→ Maintenance is the foundation of operational excellence.
🛠 Maintenance Best Practices for Maximum Efficiency
Best Practice | Why It Matters |
---|---|
Digital Maintenance Logs | Track trends, predict wear, schedule service |
Thermal Imaging Inspections | Identify insulation failures and heat leaks |
Condition-Based Cleaning | Use temperature or ΔP data to trigger cleaning |
Training Maintenance Personnel | Ensures proper techniques and faster resolution |
Integrated Efficiency Monitoring | Links performance KPIs to maintenance strategy |
→ Efficiency gains are realized when maintenance is strategic—not reactive.
In conclusion, regular maintenance is the backbone of high-performance boiler operation. It ensures that combustion, heat transfer, and control systems remain aligned, clean, and optimized. Without it, performance steadily deteriorates—driving up fuel use, emissions, and operating costs. A well-maintained system, however, performs closer to design capacity, consumes less energy, and runs more reliably. In thermal energy systems, maintenance isn’t just support—it’s an essential driver of efficiency and sustainability.
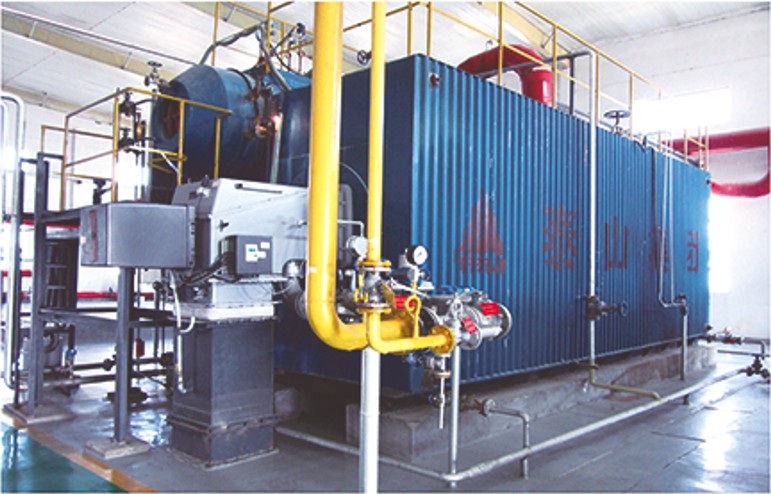
How Can Real-Time Monitoring and Boiler Automation Reduce Operational Costs?
In industrial boiler systems, energy, labor, and maintenance constitute the largest operational costs. Traditional manual monitoring methods—while still used—are often reactive, delayed, and prone to human error. As fuel prices rise and emissions limits tighten, real-time monitoring and automation have become essential tools for cost-conscious, efficiency-driven operations. These technologies transform boilers from passive systems into intelligent, self-optimizing assets, capable of adjusting to load changes, detecting anomalies, and reducing unnecessary energy consumption—all without manual intervention.
Real-time monitoring and boiler automation reduce operational costs by continuously tracking key performance indicators such as flue gas composition, fuel flow, steam output, and temperature, then automatically adjusting combustion parameters, load profiles, and cleaning schedules to maintain optimal efficiency. This prevents energy waste, reduces fuel consumption, minimizes emissions penalties, and avoids costly maintenance caused by undetected faults. Automated systems also reduce labor dependency and downtime, leading to measurable cost savings across fuel, maintenance, and compliance.
Smart boilers don’t just operate—they think, optimize, and save.
Real-time monitoring and boiler automation help reduce operational costs by maintaining optimal combustion, preventing faults, and minimizing energy waste.True
Automation continuously adjusts air-fuel ratios and load response, while real-time sensors detect issues early, cutting fuel and maintenance expenses.
🔍 Core Functions of Boiler Monitoring and Automation Systems
Feature | Purpose |
---|---|
Real-Time Sensor Data | Tracks O₂, CO, flue gas temp, steam flow, fuel input |
Combustion Control (O₂ Trim) | Adjusts dampers and burners to optimize air-fuel ratio |
Automatic Load Management | Matches fuel firing rate to steam demand |
Alarm & Fault Detection | Identifies leaks, pressure drops, scaling, and combustion drift |
Automated Cleaning (Sootblowing) | Triggers based on pressure drop or temperature thresholds |
→ These functions enable the boiler to self-optimize and prevent inefficiencies.
📊 Impact of Real-Time Monitoring on Cost Reduction
Performance Metric | Without Automation | With Automation | Savings Impact |
---|---|---|---|
Thermal Efficiency (%) | 75–80 | 85–89 | Fuel use ↓ 5–15% |
O₂ Variability (±%) | ±2.5 | ±0.5 | Air control improves |
Steam Output Stability | Fluctuates | Smooth and responsive | Load handling ↑ |
Downtime per Year (hrs) | 80–120 | <30 | Maintenance cost ↓ |
CO/NOx Compliance | Reactive | Proactive, real-time | Emissions penalty ↓ |
→ A well-automated system pays for itself in less than 2 years in most plants.
🧪 Case Study – Automation Upgrade in a 40 TPH Coal Boiler
Problem: Unstable steam output, O₂ drift, high CO (avg 180 ppm)
Manual Operation:
Efficiency ~78.5%, flue gas temp = 240°C
Operators adjusted dampers every 2 hours
Intervention:
Installed SCADA + PLC-based automation
Added real-time O₂, CO, temp sensors
Auto-tuned burners and sootblowers
Results (Post Upgrade):
Efficiency = 86.4%, flue gas temp = 185°C
CO = 55 ppm, O₂ maintained at 3.9%
Fuel savings = ~3,400 tons/year
Unplanned downtime ↓ by 70%
→ Automation enabled continuous efficiency—not just occasional optimization.
📈 Operational Cost Savings from Monitoring & Automation
Cost Area | Pre-Automation (Annual) | Post-Automation (Annual) | Reduction (%) |
---|---|---|---|
Fuel Costs | $1,200,000 | $1,035,000 | 13.75% |
Maintenance Labor | $150,000 | $105,000 | 30% |
Downtime Losses | $90,000 | $22,000 | 75.5% |
Emissions Fines | $18,000 | $0 | 100% |
→ Combined savings: over $295,000/year from real-time monitoring investment.
🔧 Technologies That Enable Smart Boiler Operation
System or Device | Role in Cost Savings |
---|---|
SCADA / HMI Dashboards | Centralized control and data logging |
PLC-Based Combustion Control | Executes air-fuel adjustments based on real-time data |
O₂ / CO / NOx Sensors | Input for combustion optimization and emissions tracking |
Steam Flow and Pressure Sensors | Adjust fuel and feedwater in real-time |
Smart Sootblowers (On-Demand) | Clean only when needed → avoids energy waste |
→ When connected, these components form an intelligent feedback loop.
🛠 Best Practices for Implementing Monitoring and Automation
Practice | Benefit |
---|---|
Calibrate Sensors Regularly | Ensures data accuracy and reliability |
Train Operators on Digital Tools | Promotes better use and trust in automation |
Integrate Energy KPIs into Dashboards | Makes performance goals visible and actionable |
Set Alarms for Efficiency Drop | Enables fast response to issues |
Benchmark Before vs. After Data | Justifies ROI and guides further improvements |
→ Smart systems still require smart oversight and disciplined use.
In conclusion, real-time monitoring and boiler automation are powerful enablers of cost reduction in thermal systems. They convert traditional reactive operations into proactive, intelligent processes that conserve fuel, stabilize output, and avoid costly failures. With automated control of combustion, sootblowing, load response, and emissions, plants achieve greater efficiency with fewer resources. In the future of energy management, automation isn’t a luxury—it’s the engine of profitability.
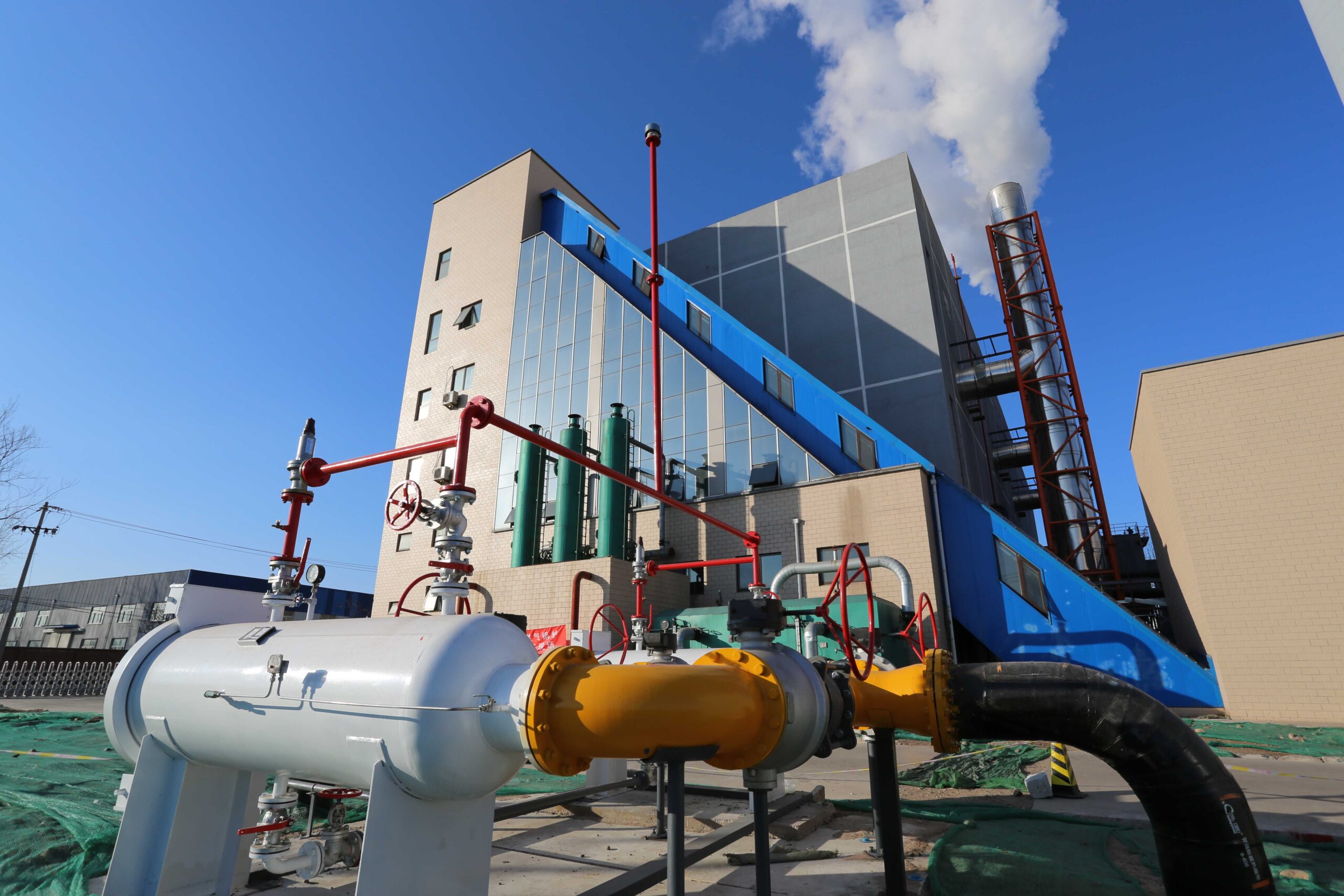
How Does Proper Load Management Prevent Cycling Losses and Inefficiency?
Boilers are most efficient when operating at or near steady-state conditions. However, many facilities experience frequent load changes due to variable process demands, poor scheduling, or undersized system components. This causes cycling—frequent starts, stops, or sharp modulation shifts—which leads to thermal stress, fuel waste, and higher emissions. Every time a boiler ramps up or shuts down, it consumes extra fuel for purging, preheating, and stabilization. Proper load management avoids these losses by matching boiler operation to demand in a planned and predictive way, maximizing uptime and minimizing energy waste.
Proper load management prevents cycling losses and inefficiency by maintaining stable boiler operation at optimal firing ranges, avoiding frequent startups, shutdowns, and rapid load swings. It achieves this by balancing process demand, using modulating burners, employing thermal storage, and integrating multiple boilers intelligently. These strategies reduce heat-up losses, eliminate purge-cycle fuel waste, and enhance overall thermal efficiency—especially in systems with variable steam loads. Fewer cycles also extend equipment life and reduce maintenance costs.
In steam systems, it’s not just how much you produce—it’s how consistently you produce it.
Proper load management minimizes cycling losses by keeping boiler operation stable and within efficient firing ranges.True
Reduced cycling lowers purge losses, thermal stress, and inefficient fuel usage during frequent ramp-ups or shutdowns.
🔍 What Are Cycling Losses and Why Do They Happen?
Cycling Event | Description | Energy Loss Mechanism |
---|---|---|
Startup / Shutdown | Boiler brought from cold or warm state to firing | Purge losses, preheat energy wasted |
Rapid Load Fluctuations | Sudden demand changes requiring burner modulation | Inefficient combustion at low turndown ratios |
Short-Cycling | Boiler turns on/off too frequently | Repeated ignition and purge fuel waste |
Thermal Soak / Cooldown | Residual heat lost after shutdown | Energy not recovered or reused |
→ Each cycle consumes energy with no useful output during ramp periods.
📏 Efficiency vs. Load Profile – The Cycling Tradeoff
Load Condition | Cycle Frequency | Efficiency (%) | Fuel Use Impact |
---|---|---|---|
Steady Full Load | Low | 87–90 | ✅ Optimal |
Modulated Partial Load | Moderate | 82–86 | ⚠ Acceptable |
Frequent Cycling | High | 70–78 | ❌ Increased fuel use |
Short-Cycle Mode | Very High | <70 | ❌ Significant waste |
→ Stable operation at 60–100% load yields highest thermal efficiency.
🧪 Case Study – Load Management in a Multi-Boiler System
System: Three 15 TPH gas-fired boilers (1 primary, 2 standby)
Issue: One boiler cycled on/off 14 times/day due to unbalanced demand
Problems Identified:
Poor load prediction
No load-sharing logic
No thermal buffer
Solution:
Implemented SCADA-based demand forecasting
Activated staggered load sharing
Installed condensate recovery tank as thermal buffer
Results:
Startup cycles reduced by 85%
Steam pressure stabilized (±0.3 bar)
Efficiency improved from 78.2% → 86.7%
Annual fuel savings: ~105,000 Nm³
→ Smarter load scheduling = less fuel burned = more consistent output.
📈 Common Causes of Load-Induced Inefficiency
Cause | Inefficiency Mechanism |
---|---|
Manual Boiler Sequencing | Non-optimized boiler activation |
Oversized Boilers for Small Loads | Long idle times, poor turndown ratios |
No Buffer Storage | Boiler must cycle to meet instantaneous load |
Unpredictable Demand Surges | Forces fast ramping and heat-up cycles |
Inadequate Burner Modulation | Cannot match partial load efficiently |
→ These result in more cycles, more purge losses, and higher fuel bills.
🔧 Load Management Strategies to Minimize Cycling
Strategy | How It Helps |
---|---|
Use Modulating Burners | Allows smooth load tracking instead of frequent on/off |
Install Boiler Sequencing Controls | Activates boilers based on efficiency/load thresholds |
Add Thermal Storage (Accumulator) | Absorbs demand spikes without cycling the boiler |
Forecast Load Patterns | Enables preemptive boiler staging |
Operate in Sweet Spot Range | Run boilers at 65–90% load for best performance |
→ Predictable load = predictable performance.
🛠 Technologies That Enable Load Optimization
Tool / System | Function |
---|---|
SCADA with Load Forecasting | Uses process data to predict steam demand |
Boiler Master Controllers | Automate startup, sequencing, and turndown control |
Condensate Recovery System | Recycles heat, buffers return loads |
Smart Burner Management Systems | Optimizes air-fuel ratio across load ranges |
Thermal Accumulators / Tanks | Absorb excess steam or store hot water |
→ Integrated systems ensure boilers respond efficiently—not reactively.
📊 Efficiency Gains from Load Management Optimization
Load Profile Improvement | Efficiency Gain (%) | Fuel Savings (%) |
---|---|---|
Reduce daily cycles by 80% | +5.5% | ~6–10% |
Modulate vs. on-off control | +3–6% | ~4–7% |
Add thermal storage (accumulator) | +4–8% | ~5–10% |
Sequenced multi-boiler control | +5–9% | ~6–12% |
→ The more dynamic your demand, the more critical load management becomes.
In conclusion, proper load management is essential for preventing cycling losses and maintaining boiler efficiency. By smoothing demand, modulating operation, and intelligently sequencing equipment, plants can reduce fuel waste, improve steam quality, and extend boiler life. With smart planning and the right tools, load becomes a controlled input—not a cause of inefficiency. In thermal systems, the best energy isn’t just clean—it’s consistently delivered.
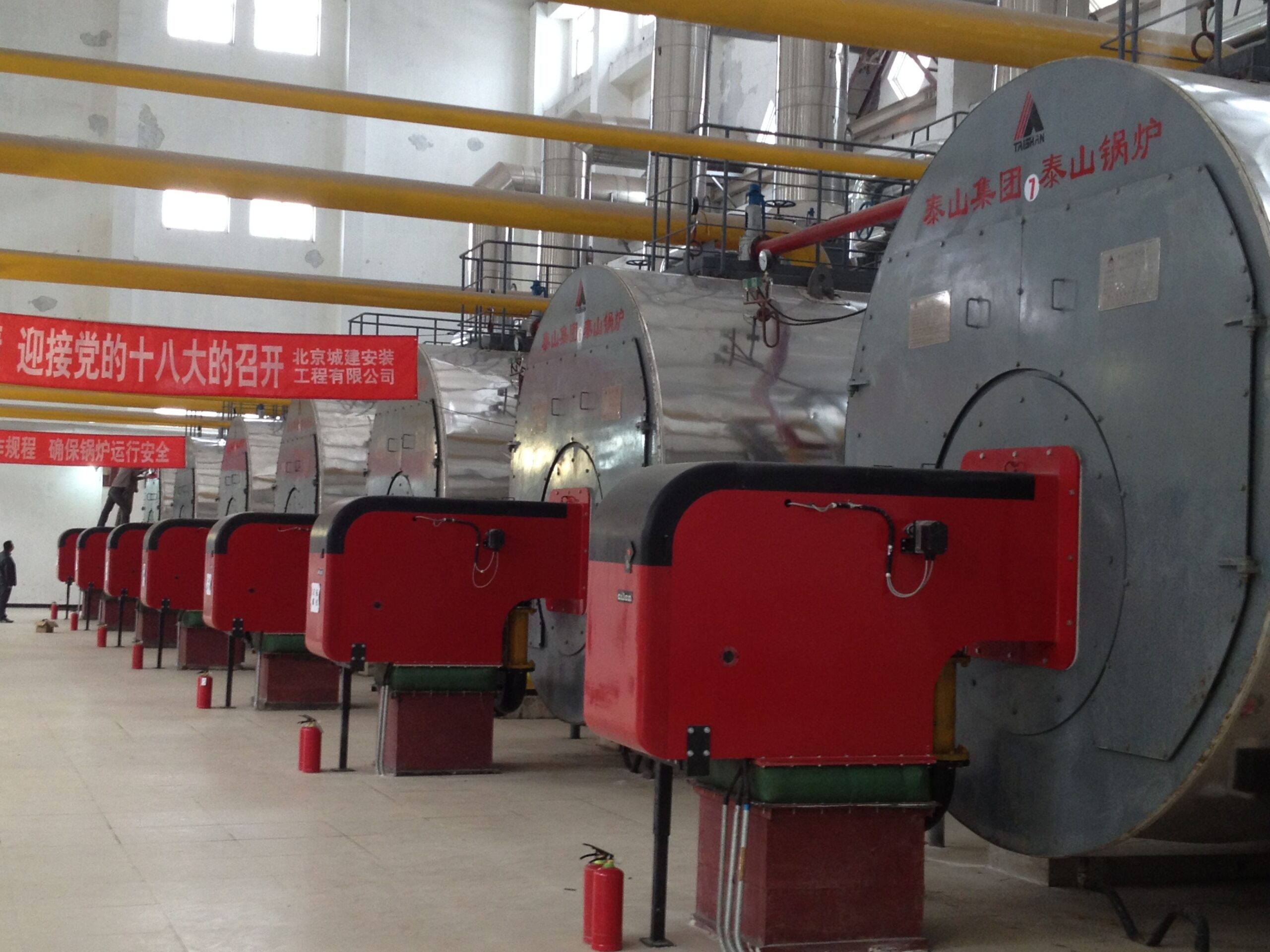
What Fuel Quality and Gas Pressure Parameters Should Be Monitored Consistently?
In gas-fired industrial boilers, consistent combustion performance and thermal efficiency depend on stable fuel quality and regulated fuel delivery pressure. Yet, these parameters are often taken for granted—leading to unnoticed inefficiencies, flame instability, and equipment wear. Even minor fluctuations in gas composition or pressure can cause combustion drift, CO formation, or temperature swings, directly impacting energy costs and emissions. To maintain optimal boiler performance, real-time monitoring of fuel quality and gas pressure is essential for both operational control and long-term reliability.
The fuel quality and gas pressure parameters that should be consistently monitored include calorific value (CV), gas composition (especially methane content), Wobbe Index, moisture, sulfur or contaminants, delivery pressure, and pressure stability. These metrics ensure consistent combustion heat input, flame stability, and air-fuel ratio control. Monitoring gas pressure prevents under- or over-firing, while fuel quality data supports real-time combustion adjustments. Together, they help maintain boiler efficiency, prevent damage, and ensure compliance with safety and emissions standards.
When it comes to combustion, you can’t control what you don’t measure—especially your fuel.
Consistent monitoring of gas quality and pressure is essential for stable and efficient combustion in industrial boilers.True
Fuel variability and pressure fluctuations can lead to incomplete combustion, flame instability, and energy losses.
🔍 Key Fuel Quality Parameters to Monitor
Parameter | Description | Why It Matters |
---|---|---|
Calorific Value (CV) | Heat energy per unit volume (e.g., MJ/Nm³) | Directly affects combustion heat release and efficiency |
Methane Content (%) | Proportion of CH₄ in natural gas | High methane = predictable flame and CV |
Wobbe Index (MJ/m³) | CV adjusted for gas density | Determines interchangeability and burner performance |
Moisture Content | Water vapor in gas | Reduces CV and promotes corrosion |
Sulfur (H₂S) & Contaminants | Reactive elements that corrode components | Affects emissions, causes acid dewpoint corrosion |
Particulate/Condensate Presence | Dust or liquids in gas stream | Blocks nozzles, erodes burners |
→ Changes in fuel chemistry affect flame shape, temperature, and emissions.
📏 Ideal Gas Quality Ranges for Stable Combustion
Parameter | Ideal Range (Natural Gas) | Notes |
---|---|---|
Calorific Value | 35–39 MJ/Nm³ | Stable output and flame characteristics |
Methane Content | ≥ 85% | High methane ensures predictable CV |
Wobbe Index | 48–52 MJ/Nm³ | Must match burner design range |
Moisture | < 1 g/Nm³ | Excess causes condensate, derates CV |
H₂S Content | < 5 ppm (typically) | Higher values require treatment |
→ Fuel deviation from these ranges requires combustion system recalibration.
🔧 Gas Pressure Parameters That Must Be Monitored
Parameter | Description | Risk if Unmonitored |
---|---|---|
Inlet Gas Pressure | Pressure entering the burner train | Too low = flame instability; too high = damage risk |
Regulated Set Pressure | Post-pressure-regulator value (e.g., 250 mbar) | Ensures safe and consistent firing |
Pressure Stability (ΔP) | Variation in gas pressure during operation | Instability leads to combustion drift |
Minimum Operating Pressure | Manufacturer-specified minimum for burner ignition | Below = flame failure, lockout |
Overpressure Trip Settings | Safety control to prevent over-firing | Prevents equipment damage and explosion |
→ Gas pressure swings must be minimized to keep the combustion system balanced.
🧪 Case Study – Pressure & Quality Monitoring in a Gas-Fired Plant
System: 30 TPH industrial gas boiler
Symptoms: Flame instability during peak shifts, CO spikes > 200 ppm
Findings:
Inlet gas pressure fluctuated between 170–280 mbar (target = 250)
Wobbe Index dropped to 45 MJ/Nm³ due to supplier blending
Air-fuel ratio stayed constant → under-combustion during low CV events
Action Taken:
Installed automated gas quality analyzer (Wobbe Index + CV)
Added mass flow control valve and pressure stabilizer
Linked analyzer output to O₂ trim control system
Result:
Flue gas O₂ stabilized at 4.2%, CO < 60 ppm
Efficiency improved from 81.4% to 88.0%
Fuel use decreased by ~8.5%
→ Real-time data closed the loop between fuel variability and combustion response.
📊 Real-Time Monitoring Benefits
Metric Monitored | Action Enabled | Efficiency or Cost Benefit |
---|---|---|
Calorific Value | Adjust fuel-air ratio on the fly | Prevents energy imbalance |
Gas Pressure | Maintain flame integrity and modulator range | Avoids low-efficiency firing or flameout |
Wobbe Index | Predicts burner performance | Ensures stable output across fuel blends |
Moisture/H₂S Sensors | Trigger alarms or filtration systems | Protects heat exchangers and burners |
→ Proper monitoring leads to better combustion control and longer equipment life.
🛠 Recommended Instruments for Fuel & Pressure Monitoring
Instrument | Measurement | Frequency |
---|---|---|
Calorimeter / Wobbe Analyzer | CV, Wobbe Index | Continuous |
Gas Chromatograph (Optional) | Full gas composition (CH₄, CO₂, H₂S) | Periodic/lab |
Inline Gas Moisture Meter | Moisture (g/Nm³) | Continuous |
Pressure Transducers / Gauges | Inlet and outlet gas pressures | Continuous |
Differential Pressure Sensors | ΔP across regulators or filters | Continuous |
→ Continuous sensors can be integrated into SCADA or burner management systems.
📈 Operational Consequences of Not Monitoring Fuel and Pressure
Oversight | Consequence | Cost Impact |
---|---|---|
Gas CV Drop Undetected | Lean flame, CO ↑, reduced steam output | Fuel waste, penalties |
Pressure Swings Ignored | Flame instability, safety shutdowns | Downtime, reliability ↓ |
High Moisture Content | Lower CV, corrosion risk | Maintenance, boiler wear |
Undetected H₂S Peaks | Tube corrosion, acid dewpoint | Heat exchanger failure |
→ Monitoring avoids hidden performance killers.
In conclusion, consistent monitoring of fuel quality and gas pressure is essential to maintain combustion efficiency, operational stability, and equipment safety in industrial boilers. Without it, plants are exposed to fuel waste, CO emissions, and safety risks. With real-time monitoring and intelligent control, boilers adapt to fuel variability, maintain efficient combustion, and reduce lifecycle costs. In thermal systems, monitoring your fuel is managing your future.
🔍 Conclusion
Lowering the operating costs of an industrial gas-fired boiler is not about one-time fixes—it’s about continuous optimization through data, maintenance, and smart control. By fine-tuning combustion, recovering waste heat, and proactively managing performance, companies can significantly reduce fuel use, emissions, and downtime. A well-optimized gas boiler is not only more cost-effective but also more compliant, reliable, and future-ready.
📞 Contact Us
💡 Need help optimizing your gas-fired boiler system? Our team provides combustion tuning, energy audits, automation integration, and maintenance planning for industrial gas boilers of all capacities.
🔹 Contact us today and start saving fuel, reducing costs, and maximizing boiler performance! 🔥📉✅
FAQ
What are the key strategies to optimize gas-fired boiler performance?
Optimization involves a combination of operational, mechanical, and control upgrades:
Regular combustion tuning and flue gas analysis
Proper burner and flame adjustment
Installation of oxygen trim controls
Use of economizers for heat recovery
Routine maintenance of heat exchanger surfaces and valves
How can combustion control systems reduce fuel consumption?
Advanced combustion controls (such as O₂ trim systems and parallel positioning) adjust fuel and air input in real time, maintaining optimal air-to-fuel ratios. This reduces excess air, improves combustion efficiency, and lowers gas usage by 5–10%.
What role do economizers play in energy savings?
Economizers recover heat from exhaust flue gases to preheat feedwater. This process improves boiler efficiency by 3–7% and lowers the fuel demand needed to generate steam or hot water.
Why is preventative maintenance important for cost optimization?
Routine cleaning of burners, heat exchanger tubes, and flue pathways prevents fouling, which can decrease heat transfer efficiency. Replacing worn-out gaskets, seals, and valves ensures stable operation and reduces unplanned downtime.
Can boiler modulation improve efficiency and reduce operating costs?
Yes. A modulating burner system adjusts fuel and air flow according to real-time steam or heat demand, avoiding inefficient on-off cycling. This leads to longer component life, consistent combustion, and significant fuel savings.
References
Boiler Energy Efficiency Improvement Guide – https://www.energy.gov
Combustion Optimization Techniques – https://www.sciencedirect.com
Industrial Boiler Controls and Automation – https://www.automation.com
Oxygen Trim and Burner Management Systems – https://www.researchgate.net
Economizer Retrofit Case Studies – https://www.bioenergyconsult.com
Gas Boiler Efficiency Trends and Maintenance – https://www.mdpi.com
Preventative Maintenance for Industrial Boilers – https://www.epa.gov
Energy Cost Reduction in Steam Systems – https://www.iea.org
Boiler Tuning and Flue Gas Analysis – https://www.energysavingtrust.org.uk
Smart Controls for Industrial Boilers – https://www.asme.org
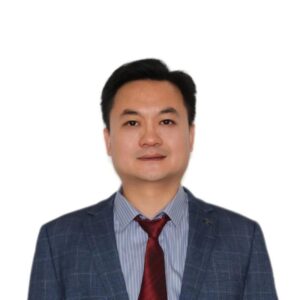